Quantum Fundamental Constants in Brighton, with Amber Shepherd, University of Sussex.
Dan: Hello, and welcome
back to the quantum divide.
This episode.
, we're taking a little bit of a
tangent away from quantum networking.
, only a slight tangent.
, we're having a really interesting
chat with Amber Sheppard.
Who's a PhD student at the
university of Sussex in the UK.
And she's.
, I've got a really interesting
experiment using quantum clocks.
, to measure the.
Delta kind of shift.
, potential shift in the fundamental
constant between the mass ratio
of the photon and the electron.
And yeah, of course we'll
dive into that in some detail.
But she's got a particular
interest in lasers.
And the kind of control that you.
You can affect with lasers
and ultimately that's.
That's how this experiment works.
And I think that's relevant really
because we use lasers a lot.
Quantum computing and quantum networking.
So I find this a fascinating conversation.
Hope you do too.
Cheers.
Thank you, Amber.
Thank you for joining the Quantum Divide.
Welcome to the podcast.
Amber: Hi, nice to be here.
Dan: Excellent.
So as we normally do, we kick off with
a bit of a, time for your background
and a bit of a bit on your story.
If you want to uh, , let us know
how you got here in the first place
and then we'll take it from there.
Amber: Yeah in some ways, I took a bit
of a weird route into where I am now.
At school, I didn't actually do
physics or chemistry at A level.
And then straight after
school, I went to art college.
And it was at that point that I
decided that science was what I
wanted to do, but I didn't have
any of the qualifications to do it.
But I was able to enroll as an external
candidate to a college in London.
And so I did all of the study at home
and then sat the exams in London.
And then I went to the University
of Sussex and I studied chemistry
there did a integrated master's and
in my final year I worked in the
astrochemistry lab at Sussex Professor
Wendy Brown's lab, that was really great.
And at that point in my final year,
I started looking around for PhDs.
And there were a few that I
found that I was interested in.
But then I started looking at physics
PhDs rather than chemistry ones.
And I found this project that I'm on now.
Um, And what really drew me to
it was they were looking for
Variations in fundamental constants.
So I met a lot of fundamental constants
in my degree and you know Everyone
assumes that they're constant that they
don't change so the idea that things
like the proton to electron mass ratio
could be changing was just Quite a
shock to read and I really wanted to
be a part of a team that was looking
into that So yeah, I applied and
Here I am doing my PhD in that group
Dan: What I like about that is the
fact that you joined college and
you suddenly realized you had to
put the handbrake on and do a 180,
And it's great to hear that's possible.
Uh, A lot of people think that.
I get, you know, once you're on that
trajectory you kind of thought to slog it
out, but that's pretty, pretty impressive.
What what made you make that decision?
Was it, was there a particular thing or
a moment that you think you can recall
that you realized, I don't want to do art
anymore, or at least I don't want to study
art and I want to go into the sciences.
Amber: Yeah, I think it was around
when I, I started doing like
interviews and applying for design
courses at universities and I think
I could feel my heart wasn't in it.
It was something I was interested
in, but I had this pull of I feel
like I really want to do science
and I'm going to miss out on that.
I'd been always interested
in science growing up.
Me and my family, we'd go to science
museums and I would watch videos
about science and read magazines
about science and all sorts.
And I think maybe when I was in
school, I didn't quite realize
that you could do that as a job.
I think I hadn't really heard
enough about scientists.
And then I did start to hear about
scientists and see more scientists.
And . Suddenly I thought, oh no, I
don't have the qualifications to do
this, but now I know that I could have
got those qualifications to do this.
And so started looking into roots in
I did want to go to like a careers,
this was when I was a teenager.
I went to some sort of careers
advice session where they said, oh,
you might really enjoy something
like engineering or science.
And.
I said oh, great.
What, What do I need qualification wise?
And I was already doing
my A levels at this point.
And they said oh you, you'd need
um, physics or something like that.
And I said I'm not doing that.
And so I thought I'd missed
the chance at that point, but
no, there was a way back in
Dan: Excellent.
Amber: to go external.
Um,
Dan: Yeah.
I guess a lot of these things aren't just
the path is given to you, it's, if you
want to make a significant change, you
have to drive that yourself, don't you?
I imagine that's what going external was
and trying to basically correct your path
Amber: yeah.
Dan: Oh, fascinating.
Thank you.
So let's dive into some
of the physics, shall we?
First of all you mentioned the
proton and neutron mass ratio.
Okay.
I, I can envisage what that is, but.
Amber: Oh yeah.
The proton To electron, mass ratio.
Dan: yeah, to the electron.
Yes.
Okay.
I wrote down neutron, but that's wrong.
Okay.
It's an, it's another
fundamental piece of an atom,
Amber: yeah.
Dan: Yeah.
Yeah.
Yeah.
I'll get away with that one.
So tell us why that interested you,
what it is, and surely there must
be a fundamental Fundamental number
that doesn't change, but it sounds
like that's not quite the case.
So yeah.
Yeah.
Amber: Yeah, so the proton to electron
mass ratio is literally the ratio between
the mass of the proton and the mass of the
electron that make up atoms and molecules.
So in my chemistry degree this was
something that I was quite familiar with
because you often use the masses of atoms
and in equations and things like that.
So, Everyone assumes in the
standard model assumes that
that these things are constant.
So standard model of physics
quite a famous model.
It doesn't quite work.
Explain everything perfectly.
Something it doesn't predict is dark
matter, for example, but something
that it says is that all of these
things should remain constant.
So things like the speed of light
and this ratio between the mass
of the proton and the electron.
But actually when you start to make
new scientific models that do explain
dark matter, those models start to
predict that these kinds of constants
may actually be changing very tiny
amounts through time and space.
So that's sort of what we're
looking into in this experiment.
It's this idea that maybe
these fundamental constants are
actually changing through time.
So we're looking at changes through
time rather than changes through space.
And we're doing that
with this quantum clock.
So, I don't know if I should
explain clocks yet or whether
Dan: Let me ask another
question first, if that's
Amber: Okay, yeah.
Dan: So that there's the, is there a
similar ratio between protons and neutrons
or neutrons and electrons and so on that?
I'd imagine these are fundamental
particles that make up atoms, right?
That they also have standard sizes and
Amber: Yeah, so if you forget about the
possible variations, the way that you look
at these things, they all have set masses.
So the proton and the neutron and the
electron, they all have their set masses.
And when you look in the periodic table,
you can see they've all got masses.
All of the atoms have masses that
have been calculated from these
sort of supposedly known masses.
And so the electron has a much smaller
mass than the proton and neutron.
And so in this sort of
traditional view, of
Dan: view.
Amber: yeah, the accepted view it's
Yeah, these things have, one proton
in one atom doesn't have a different
mass to a proton in another atom.
There's some accepted mass and then
therefore some accepted ratio between the
masses that's supposed to stay the same.
Dan: Yeah, and that's how the periodic
table is nicely laid out, isn't it?
With number of electrons is often
shown in one of the corners and,
Amber: Yeah.
Dan: Yeah.
Great, okay.
I guess we don't need to
go into the detail of that.
Most people listening to this one
know about the periodic table.
So yeah, tell us then you
mentioned quantum clocks.
How the hell has quantum, have quantum
clocks got anything to do with this
and how does it help you suspect
that there's a change in this ratio?
Amber: Okay, so like with quantum
clocks, the way they work is you've got
a transition, an energy transition in
an atom or a molecule that you know the
frequency, the energy of really well.
And then you probe this with a laser.
So it works a bit like a normal clock
where you've got some kind of oscillator,
but now instead of a pendulum, we've
got a laser, which is a light wave.
So it's oscillating like a
pendulum moving from side to side.
And now your reference, instead
of being like the hands on
your clock, it's your molecule.
So in our case, we use nitrogen.
So we've got this nitrogen molecule.
And usually if you build a clock, what
you're interested in is telling the time.
And so the idea would often
be, you know, the frequency of
this transition really well.
And you can think of
it in a couple of ways.
So frequency is the inverse of time.
You can also think of it as you've
got a wave and you can see the
distance between peaks in the wave
and this gives you some link to time.
And so usually you just know
this frequency of this laser,
this transition really well.
And so you can use it to make a really
accurate definition of one second.
But we're not interested in timekeeping.
What we want to see is whether the
frequency of this transition in our
clock actually changes over time.
So it would be a bit like, the second
getting slightly longer or slightly
shorter kind of thing in our clock.
What we would expect if
fundamental constants are
changing over time is to see this.
in like the ticking of
our clock sort of thing.
So we are, our clock will just be
sensitive to the proton to electron mass
ratio and then there are other clocks
in other experiments that are sensitive
to different fundamental constants.
So in this way we can probe one
fundamental constant and then compare
it to a clock that's sensitive to
different fundamental constants.
That's how you choose in some
ways what species to use for your
clock for this kind of experiment.
Dan: Okay, time for some
stupid questions from Dan.
First of all, you mentioned capturing
the, or measuring the wavelength.
You measure that individually
for the whole atom?
Or do you somehow measure the
wavelength individually for
the proton and the electron?
Amber: So it's for the
molecule as a whole.
So like, there's one specific transition
in nitrogen that we're looking at and
we call that our clock transition.
So Essentially what we're doing
with the nitrogen is we're
exciting it vibrationally.
So nitrogen it's a molecule, it's
two nitrogen atoms stuck together.
And it's two balls on a
spring, they can vibrate.
So what we're doing is we're
exciting that vibration.
Now Atoms and molecules, a quantum
property that they have is they
can only exist in certain energies.
So what we're doing is we're taking it
from one specific energy and that's its
most relaxed state, its ground state.
And we're taking it to this it's
the second vibrational energy level.
And it's that specific frequency that
we're looking at of that transition.
So it's a result essentially of
the vibration of the molecule.
That's what the frequency is.
Dan: And once again, the quantum harmonic
oscillator comes in, which is apparently
70 percent or 80 percent of physics
is all about an oscillator, right?
Amber: Yes, I've, since starting
my PhD in physics, I've noticed a
lot of talk of balls on springs.
It's yeah, it's, and it's, you can
often relate things to balls on springs,
it seems, and suddenly then you can
start to understand it a bit better.
Dan: Got it.
So yeah, so these different energy
states, they're the quanta, if
you like, they are the only energy
states that molecule can hold.
Amber: Yeah.
Dan: How are you exciting it?
You may have covered that already
at the beginning, but are you're
sending in a pulse from a laser.
What does that do to the?
Molecule, does it take on electrons or is
it just, it's vibrating more basically.
Amber: Yeah.
Dan: the wavelength is changing.
Amber: Yeah, so basically what's
happening is that so we have this
molecule, it's in its most relaxed state.
And as I said, yeah, it's got these
different energy states that can
exist in these very specific energies.
So we have a laser that matches
that energy essentially.
And so that's how you drive a transition.
So we have some laser we
actually use two lasers.
This is a really difficult transition to
excite, and that's by design, you want
your laser to have a very narrow line
width, so a very narrow span of energies
that will actually excite that transition,
and this is because we want really
precise measurements done with this clock.
But So because it's really difficult, we
have to use a trick and use two lasers to
get it to the state we want it to be in.
But in general, you have some
kind of radiation that matches
the energy of your transition.
And so when you give it that energy,
that's the energy level it falls
into because that's the only energy
level it can get to because of those
discrete levels that it can exist in.
So what is really changing if, if, so
the change we would expect to see if
say the proton to electron mass ratio
is changing, it's the actual energy
that we need to excite that transition.
So we'll have our laser set to some
wavelength, some frequency, and then
we'll see does it excite our molecule
into that vibrational energy level.
And if it does, we know, okay,
that's the energy of that transition.
And then we expect to see that change.
So what we would really see is that
we have to change the energy of
our laser to continue to excite the
transition is what we would see.
Dan: So one thought I had then
was around How do you control
the system so precisely?
You've got these lasers
that you're firing in.
Okay, you can just point a laser
and fire it, but surely it's,
it's way more precise than that.
You've got to ensure you've got, is it
just one molecule or is it a collection of
molecules and they all behave in that way?
Amber: Yeah so, it is, it's one molecule
what we do is We use a different laser
to knock an electron off of our molecule.
Electrons are negatively charged
so once we knock one off we have
a positive charge on our nitrogen.
And we can then use electric
fields to hold it in place in
a box that we keep a vacuum.
So we do just have a nitrogen
in what we call an ion trap.
So we are just doing the
experiment on one nitrogen.
I say this, we're not
taking this data yet.
So actually, there's quite a lot we
have to do to get everything to work.
And that's sort of what
we're working on now.
It's getting everything set up.
There are a lot of things
that have to be very precise.
In terms of our lasers.
for trying to drive that clock transition.
Yeah the frequency of that laser has to
be incredibly precise and well known.
So some things that we do, we've got
a very stable it's called a cavity.
It's basically a couple of mirrors.
And the length between
these mirrors affects what?
frequencies of light can bounce
around in that cavity and not be lost.
And we control the temperature
of this cavity really precisely
to keep it very stable.
And so we can use some electronics
to keep our laser at a stable
wavelength all the time.
And then we have something
called a frequency comb.
And this is it's like a ruler for light.
And it has, it uses GPS as a reference.
So right now in the lab, we can,
we have a little map, we can
see satellites flying above us.
we can use that to then very accurately
measure the frequency of our laser.
And that's something we've
had to set up recently.
We also have to worry about changes
in temperature and vibrations
through the floor, things
like that for our experiments.
So we have a lot of our experiments
set up on um, floating tables so that
they're not affected by vibrations
just coming from the envionment.
Dan: That's amazing to hear that
you're using traditional GPS as a
reference basically to measure something
that's on the nanoscale, basically.
Uh, Yeah, these micro cavities keep
coming up in interviews that I have.
Are they used here in the same way that
they would be used with a quantum dot
to emit photons of a particular type?
They're there to capture what's coming
out of the laser and point them in
a particular direction or get them
to interact with something else.
Amber: the cavities are
sort of part of the laser.
So The laser light comes out of
one of the mirrors in the cavity.
What the cavity does is it selects
for the kind of light that we want.
So what's happening inside the cavity
is the light is getting amplified and
it's like the light that survives in
the cavity is the light that is going
to get amplified as it passes through
something like a laser crystal or
whatever we're using to amplify the light.
Like we sit that material
inside the cavity, the light
bounces backwards and forwards.
And then light that's of the
wrong type, so something that
comes out at a different angle.
It's going to be lost, it's
not going to bounce backwards
and forwards and be amplified.
And so what we're using the cavity
for is to select the kind of, yeah,
the kind of light that we want.
And then only certain wavelengths
of light will exist in that
cavity depending on its length.
And so we can also use the cavity
to select for the wavelength
that we want from our light.
And then what comes out is
this amplified light that is
exactly how we want it to be.
And all of the photons are identical
which is useful for our experiments.
We need all of the lights
to be of the same color.
We need it to all be traveling together.
Dan: Okay.
Yeah.
Fascinating.
Look, I want to come on more to
focus on lasers a little bit.
But before that I just wanted to
ask you, we're still calling this
device a quantum, the quantum clock.
But you're not using
it as a clock, really.
Have you thought about giving it a
different name or maybe you've got a
nickname for it that's something else
that describes what you're doing better?
Amber: We've been trying to come
up with maybe like an acronym for
the experiment because some people
do that, but we haven't thought
of anything good enough yet.
But we tend to call it a clock because
in some ways that is the technology and
it's because these things are so precise,
it's why we've picked them, I guess.
we still do tend to call them the clock.
We call it different things.
So we called the lab that we work in
the molecular lab because everyone else
is using atoms in our research group.
And we'll sometimes call it the nitrogen
trap or something like that, which but
in general, we tend to call it a clock.
Yeah.
But no, we're not
telling the time with it.
But I guess we've taken that
technology from clocks because
of some of the features of them.
we're using a clock, but
to do something else.
Dan: Yeah, it's still a
measurement, isn't it?
Whether it's, it's the time and the, and
the wavelength and the distance and so on.
It's all related.
I like nitrogen trap.
That's quite good, but that's very
different to in the quantum world.
We've got ion traps and they tend to trap
very I don't know, very specific elements.
I think they use either calcium or
ytterbium, for uh, A trapped ion.
Why?
I know nitrogen is obviously a
much simpler molecule because
it's, and therefore you can
use it to do this measurement.
Is that the main reason why you use it?
Amber: What we have an ion
trap, just where you would trap
calcium and things like that.
We actually do trap calcium.
So nitrogen, it's got some sort
of advantages and disadvantages.
So the advantages are, it's a
molecule, which means it can vibrate.
So calcium doesn't have vibrational
energy levels and vibrational energy
levels are sensitive to the proton
to electron mass ratio, which is a
big reason for choosing a molecule.
Again, you can think about
the balls on the spring.
It makes sense that a vibrational
energy is going to depend on masses.
that's one reason why nitrogen,
just because it has the transition
that we need to actually probe
the thing that we're looking for.
But another nice thing about
nitrogen, so it's, it's symmetrical.
And this means that it has a
selection rule that says That it's
immune to certain excitations.
What we find is that our nitrogen
isn't affected by black body
radiation and other sources of noise
that we can't control in the lab.
Like those things are going to be
there and that could be a problem.
If it could change the state of our
nitrogen that's then an issue because
we're trying to excite a very specific.
energy transition.
And if something comes in and
destroys our state, we're now in
some different starting point and
we don't know where we're going.
But yeah, the, because of the symmetry
of nitrogen, we don't have that problem.
So it does have some really nice benefits,
just makes it nicer to work with.
But the disadvantage is being
a molecule when it comes to
the ion trap side of things.
There are some extra
steps we have to take.
So this brings me on to why we
have to trap calcium as well.
Something you have to do with
ions in an ion trap is you've
got to get them really cold.
If they're hot, they're moving
around a load and they might escape.
So we use lasers to do that.
What we do is we basically send in
a laser, it has some momentum, and
it gives our ion a bit of a shove.
So it can slow down its motion
in that way and cool it down.
The way that we make this sort of better
is that we set it up so the ion is only
going to, or is mostly going to interact.
With a laser that it is moving towards
because you can imagine if it interacts
with a laser That it's moving away from
it's getting heated because now it's just
speeding it up in the direction We don't
want it to go So the way that we get this
to work is we use the Doppler effect.
So Just like the sound waves Something
with a siren drives past you and
you hear the pitch change and that's
because from where you are the sound
waves are essentially changing their
frequency or you're experiencing
the frequency different as it moves.
And the same happens with light, so
if it's moving towards a laser it
sees essentially a different color of
that laser than if it's moving away.
And so we make our laser a bit more red,
we red detune it and then this means that
It only sees the correct energy to go
to that discrete level when it's moving
towards the laser rather than away.
Dan: methodology used to trap
neutral atoms as well, right?
The, this kind of yeah.
Throwing in energy you'd think
would excite the whole system.
But if it's, if the
directions are all correct.
Then you've got this, not a cancellation
of energy, but it's just using it to
slow it down as opposed to speed it up.
It's fascinating that there's
this level of control.
Amber: it sounds really weird
to say you're heating you're
cooling down an ion with a laser.
You think of lasers, yeah,
as heating things up.
The way that this, tends to work
is that you have some kind of cycle
in the energy levels that you're
always ending up in the same state.
But we can't do that with nitrogen.
One issue is that molecules, they have
very complicated energy level structures
compared to atoms, and that's because they
have all these rotations and vibrations.
So we can't actually do this
with molecules because what would
happen is it's just going to fall
into some other state, and again
that's then an issue for us.
So that's why we have calcium we actually
cool down the calcium, not the nitrogen,
and then the calcium cools down the
nitrogen for us in the trap because they
share some kind of motion in that trap.
Dan: right.
Yeah, just if you thought it
wasn't complicated enough, add
another layer of complexity to get
around and work around a problem.
Amber: Yeah this is the
thing with molecules.
There are some really great things
about them, but there are some
things to consider, some things
that we can't do with them in
ion traps that we can with atoms.
Dan: Okay.
Before we go on to lasers, cause I
know there's a whole bunch of questions
there I did say, I wanted to ask you
about the dark matter measurement.
You've described the system very well.
But what is it that you're
going to be looking for?
It's changes in that frequency
in the transition, but what's
that going to tell you?
Amber: so there's a couple
of things that we could see.
So that transition, as I said, has some
dependence on the proton to electron mass
ratio as in the energy of that transition.
would change if the proton to
electron mass ratio changes.
So if we see some change in
that frequency over time, that
could tell us that fundamental
constants are changing over time.
So that's one thing that we could find.
The thing with dark matter is that what we
might expect to see is if some dark matter
interacts with our nitrogen we might see
a more temporary change in that frequency.
So it would briefly affect
that, that energy level and that
nitrogen just while they interact.
And so we're going to see something
different there rather than something
that we see over a long amount of time.
What we would then look for is the same
temporary change in frequency in other
clocks in sort of our network of clocks.
So there are other experiments with
different clocks that have different
sensitivities to fundamental constants.
And we could then compare data because
of course, you do have to be careful of.
other things briefly affecting
your experiment, so it is better
to be able to see the same thing
happen across different experiments.
Dan: Yeah.
Better empirical evidence
with collaborators.
When you described the dark matter
affecting the nitrogen, I imagined what I
read about dark matter and evidence of it
is the gravitational lensing around stars
and systems in cosmology and astronomy.
Is that, so is that the same
thing that's happening down at
the molecular level in a way?
Amber: The thing with dark matter it
doesn't interact with light and it only
weakly interacts with regular matter.
So with the gravitational lensing
we're sort of inferring it's there.
By looking at how I think we're
looking at the light, right?
And how it's affected by the gravity,
which so I think it's a bit different.
So it's not it's interacting
with the matter, I think.
I mean, This is a little
bit further away from um,
yeah, but basically what it's
doing, it is interacting, I think
with the nitrogen and it's causing
it to change color very slightly.
So when, when you're looking at the
gravitational lensing, you're sort
of looking at you've got some mass
up there, it's got some effect on
gravity and that has some effect
on light and we can see the light.
We can't see.
light directly interacting with dark
matter but we can see how the light
is like bending in a funny way.
Dan: Yeah.
Amber: With our experiment, the
dark matter is actually interacting
with regular matter and what
we're seeing is some change in
the energy levels of the nitrogen.
It's a bit different in that we're
actually seeing an interaction of dark
matter and regular matter in a way
rather than something, some effect,
some effects that we're inferring from.
We are, we're not, we're still
not seeing the dark matter,
but we've, it's invisible,
Dan: It's still in inference.
Yeah.
The, The reason why I said it
was, I think, because we're
measuring a wavelength, which
is, light has a wavelength.
So perhaps I made the connection
there, but I understand it's
a different type of thing
Amber: Yeah.
So I guess, yeah, gravitational lensing,
it's whatever light is floating around
and we're just seeing how it's moving.
Dan: Hmm.
Amber: what we're looking at, the
light is the light we're sending in.
And what's actually changing
is the matter itself.
So the dark matter is coming in
and it's changing the energies
that our molecule would sit in.
Dan: It's changing how
boingy the spring is.
Amber: Yeah, kind of, yeah, yeah.
So in one case, you're seeing it bend
some random light and it's not really,
it's not interacting with that light.
The light is just being affected
by gravity, which is being
affected by the dark matter.
But in our experiment, it's a bit more
direct because it is actually changing
the energy levels in our nitrogen
and then we can measure that instead.
Dan: Cool.
And just to wrap this up in
terms of timeline and, when
will you start measuring?
How long will you leave it running for?
Are you expecting to see some results?
If you are, will you get a Nobel prize?
Amber: So it's still a long way off.
There's a lot of things we
really need to get right.
And so we're very much on the
setup phase at the moment.
But once the experiment is running and
that may be after my time as in my time
in the group but We would then be looking
for a very long time, like years scale.
So something, the longer you can
look, the more sure you're going
to be whether it's changing or not.
We may see something or we may see
nothing and either could be considered
good in a way, so people have already
done experiments like this, and
they're not seeing any changes, and you
don't at that point say, okay, so the
fundamental constants aren't changing.
You say, we now know that they're
not changing by this amount,
because we would have seen it.
And so you can keep pushing the
constraints down and saying, okay,
it at least doesn't change this
much over this amount of time.
So we're trying to push that boundary and
look at a a different level of change.
So it's being constrained to one value,
this, these fundamental constants.
And then we're trying
to push that boundary.
further.
If we did see something, if we
saw some sign of dark matter or
fundamental constants changing,
that would be a really big deal.
Don't know if there
would be a Nobel Prize.
I haven't
Dan: I'm jesting, of course.
Just wondering if it's like the LIGO
experiment where we thought we'll turn
this thing on and we won't see much.
And.
Once it got running, all of a sudden we
realized there were all these pulsars
and all these binary systems sending
out gravitational waves all the time.
But I'm guessing it's more likely
that you're going to switch this
on and it's not going to change
for a long time because we've been
relying on the fact these constants
are constant for us, for our whole
science, for our standard model, right?
Amber: Yeah, so these
changes would be so tiny.
And at first like, say after a week
of running this experiment, I don't
think we're going to see anything.
Even if they were changing you
need to collect this data over
time because you can imagine if
something is changing by tiny amounts.
So you've got some line that
has, A really tiny gradient.
You're not going to be able to
say that that's there until you
can watch it for a very long time.
It would take a lot of data.
So I don't think, I don't think we
would run it for a short time and
then say, ah, yes, we've got, and, but
we also, an exciting thing about it.
is that we also don't know for sure what
we'll see like, will it be just like
a gradual drift or will it oscillate?
That's another possibility
that it's oscillating in time.
And this information could be really
useful for people who are making
these different models that do predict
changes in fundamental constants because
they can then use that information.
to tell us something about, say,
dark matter, because different models
predict different kinds of changes in
fundamental constants, so that's another
thing like, we don't know completely
what we're looking for, either.
What kind of change.
Dan: Yeah.
Fascinating.
Honestly, it really is.
So let's bring it back to like the, a
little bit to the traditional IT world
where we use lasers for different things.
Obviously I'm not referring to the,
you know, we've all seen Goldfinger
and that, that that famous scene where
he says, do you expect me to talk?
But no, I'm not talking about that.
I'm talking about like traditional
world of transceivers and the way we
use light pulses to encode information.
We'll get to that, first of all, what
are the different types of lasers?
What are the use cases for lasers
and which one does this fall into?
You mentioned laser cooling, but I think
there's more than that, isn't there?
Amber: Yeah so, we use lasers for
a few things in our experiment,
but they're used for all sorts in
everyday life and other experiments.
Yeah, we have our laser that's
going to probe that clock transition
and the lasers for cooling.
We also use lasers for
ionizing our nitrogen.
So we use it to use them
to knock an electron off.
And we do actually need for our, for
our molecules, so sometimes you can use
lasers that are so there's different types
of lasers, continuous wave and pulsed.
And sometimes uh, you can
use continuous wave lasers.
So these are lasers where the
light is just constantly emitting.
And you can use those for
ionizing in some cases.
But for us we need our nitrogen
to be in a very specific state.
So we use a slightly different ionization
process, and it requires two photons
to interact with our molecule at once.
And this is quite an
unlikely process to happen.
And We need really high
intensities to sort of overcome the
unlikeliness of what we're doing.
And so we use these pulse lasers.
And what you're doing, instead
of the light just constantly
being on, you constrict it to
these little packets of time.
And so what you're doing is, rather
than constant lower power, you
have these bursts of higher power.
And so we can get higher
intensities of our laser and
so do more unlikely processes.
This does make the lasers a bit
more dangerous to work with because
suddenly they can burn things and then
when I say dangerous to me because
they could burn my eyes, they could
burn my skin, but also dangerous
to our equipment and they can start
breaking mirrors and things like that.
You, you have to start taking
precautions wearing goggles and also
buying slightly different mirrors
and optics so that they're not
going to get scorched by your laser.
Dan: So we are talking about
Goldfinger style then, really?
Amber: yeah lasers are used for cutting
things, so a day to day life use laser
cutting And these things, I can't
remember the exact temperatures, but
they get to extremely high temperatures
to materials as they are cut.
Lasers can be very dangerous things.
And especially to eyes,
because, of course, your eyes
are designed to absorb light.
And so lasers can do
some really nasty damage
Dan: Yeah, just reminds me actually of
the new laser weapon that they're going
to be putting on all the naval ships.
I can't remember the name of it,
it's called Dragon Eye or Dragon
Fire or something cool like that.
But You know, these are like replacing
kinetic weapons which because
of the precision and the power
they've got, that's pretty amazing.
Amber: Yeah, and I mean, that
can be the danger of them.
So the other day I was looking
for the news and it suggested
to me BBC News lasers.
And so I clicked on it thinking it would
be some interesting science to read, but
instead it was lots of articles about
people who have got in trouble for shining
lasers at like helicopters and planes.
And the thing is, I think people
don't always realize actually that
these things are quite dangerous.
I mean, They can get all the way to a
plane and cause quite a lot of damage.
There's a lot of restrictions
on buying and selling lasers.
And there are different laws on
lasers in different countries.
So yeah, they can be quite dangerous.
So
Dan: again.
I was talking about pulses and.
For one use case, and you were talking
about pulses in your kind of world,
in quantum networking, we also talk
a lot about single photon sources.
And I guess that's what you're
doing with these pulses.
You're trying to, is it the same thing?
Like is a quantum dot in a cavity, which
is a single photon source, is that somehow
controlled to create pulses so small that
you end up with one photon inside them?
It's
Amber: it's a bit different, although
that is one technique that people are.
try to use to create single photon
sources, rather than restricting
the time of the laser, just
turning the power right down.
I think I read about that, so
that suddenly your probability of
having one photon is far greater
than having more than one photon.
But for us, it's a bit different.
Essentially, let's say you have
some number of photons that's coming
out of a continuous wave laser.
If you now start to push those photons
into little into shorter spaces
of time, you now have more photons
in that pulse, but then no photons
between that and the next pulse.
Anything, you have more photons
in that pulse and that's what
gives it that really high energy.
So we, we want lots of photons because
we have this really unlikely process.
So just the more we throw at it, the
more the probability goes up that
we're going to actually drive it.
We sort of do it to get
more photons than less.
But if we were to turn the power down
and go, not the other way, but make our,
lasers less powerful, then you might start
to get more towards the single photon.
But yeah, as you say, it's
different technologies that are
Dan: Okay, so it's the other end
of the spectrum, so to speak.
Excuse the pun, but you're cranking
up the power within that pulse.
Amber: yeah, we're getting,
yeah, higher intensities there.
Dan: how many packets of
time is it till lunchtime?
That's all I'm thinking about.
No, not at all.
Uh, Yeah.
So traditional network transceivers,
which send pulses of light with data
encoded, and how are they different
to the pulses that you're using
to crank up the number of photons?
Is it just that there's no encoding
of data essentially, and it's
about, like you said, increasing the
probability of the transition happening.
Amber: Yeah.
So for us, our pulses, there's
there's no kind of pattern in
them or anything like that.
There's no data encoded into that light.
It's they're just evenly spaced
pulses where we're essentially just
throwing some photons at it and
then throwing some photons at it.
And that's what we're
doing with our pulses.
We do with transceivers we do have a
sort of similarity in our lab in that we
also transport light over like fibers.
So I think the idea with transceivers
is you're you're using light to send
data because it can travel at the
speed of light, so you, it's sort of
as fast as you can get your data to go.
And then the transceivers are there
to convert the light into some kind
of electronic signal and then turn
the electronic signal back into light.
I guess this is quite important
because you are going to attenuate,
lose your signal a bit in the fiber.
For us, we are not sending our
light over nearly as far of a
distance, but we do actually use
fiber optic tape cables in our lab.
So we also have to transport light around.
And it is actually, I assume very similar.
technology, I don't know, it won't
be exactly the same kind of fiber
I'm sure, but we also do that, but we
don't need the transceivers because
we're not losing enough of our
Dan: and you don't need to move
it to an electrical signal either,
Amber: no we actually do also use, so
something you can do is have something
like a photodiode, so we often use that
to monitor our lights, so for example
maybe you have two different pulse lasers
and you want the pulses to occur at
the same time or something like that.
You can see a pulse on these photodiodes
so basically a photon hits a photodiode
and then it results in some kind of
electronic signal and you can look up at
that on a computer and see the pulses.
So we do use that kind of technology but
again yeah for a different kind of thing.
We're not taking any data out of
the light that is encoded into the
light, we actually want to learn
something about the light itself.
Dan: Okay, very nice.
So there's a few different kind of
questions that I wanted to bring.
First of all, I know you're in your
PhD, so you may not have released any
papers yet, but are there some papers
you've worked on or contributed to
or seen developed at the university
perhaps that you'd like to highlight?
Amber: At the moment uh, yeah I've,
I've not worked on any papers in my PhD.
But hopefully soon, so we're currently,
I think there's been a lot of set up
As we've discussed, there's a lot we
need to get right about this experiment
and so we've been getting everything
ready but hopefully we're at the point
now where we'll start getting a bit
of data, not data to try and find dark
matter, but data where we can test some
of our lasers and some of our processes.
I've been working a lot with a paper from
our group recently where someone called
Amy Gardner, who worked on the molecular
experiment I think several years ago she
did what I'm doing right now, where she
had some lasers, these pulse lasers, and
she would send the lasers into a vacuum
chamber, and then send in some nitrogen,
and she was testing them out, basically,
and What happens is you create some ions
they're positively charged, and then
you use an electric field to accelerate
the ions up towards the detector.
And the reason that we do this is
just to characterize the lasers,
work out exactly what wavelength we
need to do the ionization and also
test out the lasers themselves.
So I am now trying to revive
that experiment with the
new lasers that I've built.
So she was using something
called dye lasers.
They're lasers where you use
a liquid with a dye in it to
tune the color of your laser.
The thing about dye lasers that isn't
good for us is that they have a very
broad line width, which means that.
their output essentially contains
quite a lot of different frequencies.
And this means that, for some number
of pro or photons, sorry not protons.
You have only a small amount of
light with the right energy for
that ionization and the rest of it
is just stuff that's not useful.
And so you've got to turn up
the energy more because you've
got to get more light in that.
useful range of energies.
And then, this is inefficient, and it
causes damage to our equipment and so
I've set up some lasers that are a lot
narrower but they need to be tested now.
And that's the paper I've been reading
over and over again at the moment,
because I'm, Literally, I'm trying
to copy what she did, but with these
new lasers, to see if they're better.
Dan: Nice.
That sounds like there's
been, people working on.
things related to your experiment
historically in the same lab,
and it's all been building up
to this work that you're doing.
Is it the first time this, I
forgot to ask at the beginning,
is this the first time that this
type of experiment has been done?
Amber: So no um, people have done this
experiment before, but the idea is
someone will push the constraints of
these values so they'll be able to say
to this level, they're not changing, the
fundamental constants aren't changing.
And then what you do is you go away and
you make whatever improvements you can.
So quantum clocks are always
being improved, they're finding
better species to use and better
ways to set up the clocks.
And so as the technology gets better
you can then run the experiment
again with the new technology and
see now to some better level of
precision whether they're changing.
It's the first time perhaps that someone
has tested them to this level, but
people have done experiments like this
Dan: Oh, that's cool that you, yeah,
it's like an incremental increase
in some factor every time whether
it be accuracy or stability or
Amber: Yeah.
Dan: yeah.
Yeah, okay, sticking with papers is there
another paper you'd like to highlight,
specifically in the quantum domain?
Is there an influential piece of
work or something that has been
particularly interesting to you?
Amber: I did want to bring up, so there's
a paper I hope this counts as quantum.
I mean, Lasers are, are quantum, right?
Um,
Dan: yeah,
Yeah.
Amber: So actually the first ever paper
written about the invention of the laser.
So it was in the 60s.
And some researchers invented the laser.
And they wrote a paper about it.
I think one or two page paper.
And they sent it to a journal and
it was rejected, which I find really
interesting because of course now
loads of people are using lasers
for loads of different things.
But at the time it was called like
a solution looking for a question.
That's what some people called
the laser because they'd invented
this thing, but it wasn't clear
yet exactly how it would be used.
And in the paper they detail sort
of what they'd done, what they'd
set up, and what they'd seen.
And it was, yeah, the first
creation of something like a laser.
But I really like the story
of it because it's similar to
us looking for dark matter.
Like people sometimes ask,
why look for dark matter?
You know, what, What are
you going to do with it?
What, how is it going to
help people kind of thing?
And I think it's good to think about the
invention of the laser because at the
time people were like what is this for?
But now we use it, not just in
science, but it's used in medicine.
And it's used in entertainment.
And in laser cutting, it's a really useful
tool and this is the thing you've got to
kind of go for the interesting physics
and get a better understanding of it
and then you start to find uses for it.
So I really like that paper and
I think it's really interesting
that it was rejected at first
because, of course, lasers now are
Dan: Oh, they're everywhere yeah, yeah.
And that's one of the things
that, People don't like about the
scientific process sometimes, right?
The, or the industry, if you like,
is that sometimes this happens.
I think there are quite a few seminal
pieces of work that were rejected
first time, including Peter Higgs's
first model back in the sixties.
And I also heard about Enrico Fermi's
weak interaction paper, which goes
way back to the 1930s, I think.
But these are things that were
rejected from a magazine for some
reason in fact, rejected from nature
for being too removed from reality.
Amber: Wow.
Yeah, I mean, it often takes
a while to see the effects of
a discovery in science, right?
And because these things need to get
useful and they need to start to integrate
into people's experiments as well.
It takes a while for people to
set these things up and find
all the new uses for them.
Dan: Yeah, exactly.
So what's your vision for the future
when it comes to either lasers or
quantum clocks or quantum computing
or, something in that domain?
Amber: Yeah I think, with what we're doing
I think the future is trying to understand
dark matter better and, looking at, say,
the example of the laser, there may be
some interesting uses and interesting
technologies that come from that.
Quantum technology is a
really exciting field.
There's A lot going on there right now.
So I don't know what quantum
technologies will come next,
but I'm excited to find out.
I think that as we improve, like our ion
traps and our lasers and our technologies,
it will be really interesting to see
Especially with quantum computing
which isn't my field, but I hear a lot
about it and it's interesting to see.
So one of the issues with quantum
computing is trying to make it scalable
because, our experiments with ion traps
often take up whole rooms or more.
So to see how people are going to make
those computers as small as possible is.
It's going to be really cool
yeah, things are already being
done actually here at Sussex.
There's some interesting designs
for making quantum computers smaller
but yeah, I just wonder where
people can push that, and that'll
be an interesting thing to watch.
Dan: Yeah.
I mean, Miniaturization has been
around for a long time, right?
Just look at the transistor and
normal, silicon computer chips.
But I guess it's like, there's another,
Another stage of miniaturization that has
to happen now because we're controlling
atomic or photonic matter or elements.
When you, you need vacuums,
you need cooling, you need
very precise laser systems.
It's it's another whole world
of miniaturization, isn't it?
Even though you, because you're, even
though you're controlling things down
at the most miniature level, trying
to miniaturize the control systems, I
think is the biggest Biggest challenge.
Amber: Yeah, there's so much
that goes into, to these systems
we've got a project in our group.
building a miniature quantum clock and
yeah, I mean, you're trying to take
something that takes up a whole table
in the lab and put it into a little box.
And yeah, as you say, you need vacuum
systems and lasers and even lasers We
have our laser light and then we need
loads of different optics to control it.
So things like mirrors to guide it around,
but we use things called wave plates.
They rotate the light things called AOMs,
which can slightly detune the light.
It's like we need all these other
tools and they're quite big as well.
And so trying to.
find ways to make those things smaller
and have it possible to align the
laser through them, but have them
take up as little space as possible.
There's so many challenges.
Dan: Oh yeah, definitely.
Lots of work to do.
So yeah, just to wrap up then um,
tell us what do you do to wind down?
Science seems to be like a heavy
focus for you, like it is most people
I speak to, but have you got any
interesting insights into what you
do to get away from science or get at
least switch off from it a little bit?
Amber: So actually, when I started my
PhDs one of my colleagues suggested
to me that I take up something
that I always make sure that I do.
Like, She, she plays hockey, for
example around that time I started
taking singing lessons, so I do that
once every couple of months maybe, and
then in between I'm like practicing
my singing, things like that that's
my thing that I try and do regularly.
I like, I still like art even
though I didn't pursue it at
university, I still like it.
Doing drawing, writing short stories, and
making short films, and things like that.
And then often after work, to be
honest, I go home and I watch TV,
watch films, play video games.
So that sort of thing helps me wind down.
Dan: Yeah.
Yeah.
Where do you think the
singing is going to take you?
Are you?
Is it, is it just for fun at the moment
or are you planning to record something?
Amber: At the moment, just for
fun, I thought I might join like a
local choir or something like that.
But Yeah who knows?
Dan: Excellent.
Thank you very much.
We'll wrap it up.
Thanks for the discussion and the lesson.
Amber, really appreciate it.
Amber: Thank you for having
me on, it's been great.
Dan: Superb.
Bye for now.
Amber: Bye.
Dan: I'd like to take this moment to
thank you for listening to the podcast.
Quantum networking is such a broad domain
especially considering the breadth of
quantum physics and quantum computing all
as an undercurrent easily to get sucked
into So much is still in the research
realm which can make it really tough for
a curious IT guy to know where to start.
So hit subscribe or follow me on your
podcast platform and I'll do my best
to bring you more prevalent topics
in the world of quantum networking.
Spread the word.
It would really help us out
Creators and Guests
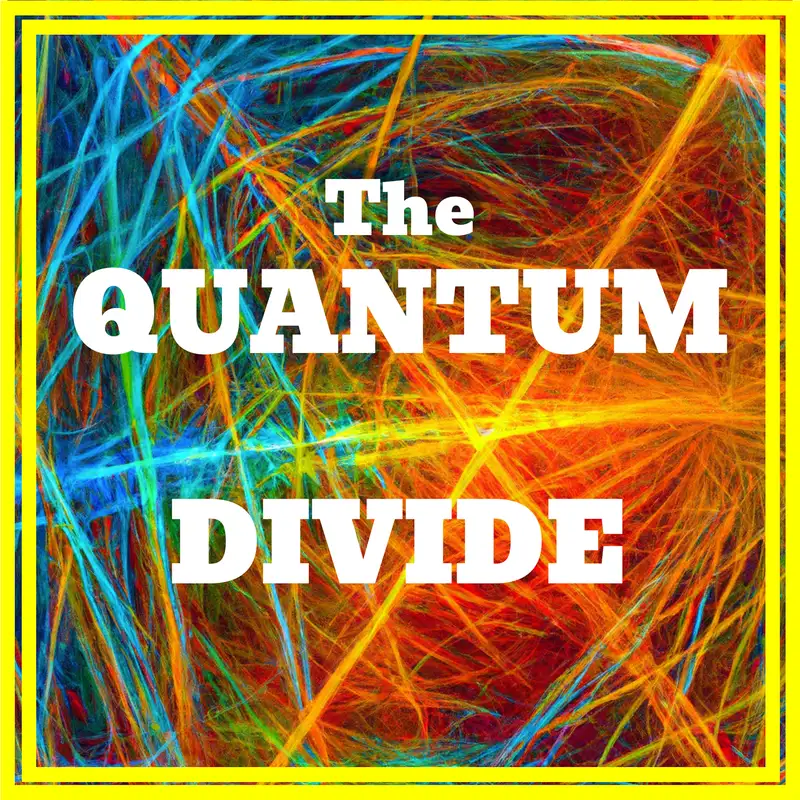