Quantum transduction and piezo-optomechanics, with Rob Stockill, QphoX.
Dan: Hello.
Welcome back to the Quantum Divide.
Thank you for tuning in once again.
This time, we're going to have a look
at . I would say the hardware at a
particular point in what would make up.
Uh, quantum network.
I like to think of these as
quantum network interface cards.
Essentially, these are the
devices which are going to sit
between the optical domain.
And the microwave., domain.
Inside a cooled.
Quantum computer.
And this is going to be really exciting
to explore this, because this is right
at the cutting edge of development
and commercialization of quantum.
information science.
So the company I'm interviewing
this time round is QPhoX and
my guest is Robert Stockill.
Rob is the co-founder and CTO of QPhoX.
I hope you enjoy it.
Alright, then.
Let's go.
Rob Stockill, an Englishman in the Delft.
How are you doing?
Rob: Hello.
I'm very well, thanks.
I'm very well.
Thank you for, having me
join you guys this afternoon.
Dan: Yeah, thanks for joining us.
Yeah, let's get started.
Why don't you give us a bit of an
overview of your background, how
you got into Quantum, how you, the
inception of QPhoX, if you like,
and then we can go from there.
Rob: Yeah, of course.
Yeah, so personally, I, my route into
Quantum is yeah, through academia.
I started working in the field
about um, 12 or so years ago
physics undergrad at Oxford.
And then started on doing some really
interesting projects working on actually.
At the beginning, trapping ions.
That was my first kind of in to
experimental quantum physics and
then moving into quantum technology.
Then I moved on to the PhD at the
at Cambridge university, working on
self-assembled quantum dots looking
at how you can basically put the
spins of electrons confined in
these systems into entangled states
and distribute bell states through
photons to interact with these spins.
But then I went over to Delft
actually as a postdoc to work
on this field of optomechanics.
So basically the interaction between
light and mechanical vibrations.
And then once I got into Delft,
there was a specific application
of this field of optomechanics,
which is building of quantum
transducers, which is really a, um.
, specific use of this interaction to
then take the interaction between the
lights and the mechanics, and then
use it to convert optical photons
into microwave photons and vice versa.
So we were working on this, I was working
on this with the now CEO of QPhoX.
Simon Groblecker.
He was my PI.
I was a postdoc.
We were working on this in the lab
doing kind of early days research,
figuring out what sort of materials
we can build these devices out of,
how can we measure how well they work?
And then come 2020 to early 2021, we
were like, we started seeing that really
it's time to look at being serious
about commercializing this technology.
So actually taking this application
and yeah, commercializing it,
fully, developing it from just
the much further than you could
do in a academic research lab.
So we went out and of course.
To begin with, it's an investment game.
You can't start this
company from just scratch.
There's a a lot of infrastructure
and equipment and people needed to
really push this technology out.
But we were lucky enough to find some
really motivating, enthusiastic investors.
So 2021 about May we really kicked
off the company properly, QPhoX with,
there were six of us at the beginning.
Now in early 2024, there's
twenty-one people in the company.
So it's grown quite a lot.
And we've, yeah, just it's been a
kind of a wild two and a half years
of getting everything going and really
pushing this technology forward, both
in its core performance, but also
its applications with end customers.
Dan: Yeah, I guess it's a factor
of combination of , researching and
learning the technology and at the
same time the start of the need,
potential need for it from the
development of quantum computing, right?
All these things have gotta be lined up.
Yeah.
Rob: Yeah.
Exactly.
Exactly.
And it's one of these technologies,
this quantum but it's very much if
it was really there as a product,
it would be in huge demand.
It's the sort of thing which the
developers of quantum computers
they want it, they want to ASAP.
But I'm finding and matching those
development timelines is always Yeah.
Key.
Really.
Dan: Okay.
So let me start with the question.
Why you called QPhoX?
I've tried to guess a few times, but.
Rob: What your main, you don't wanna
tell me what your main guess is?
Dan: I was thinking quantum
photonic OR photon, and then X
is the transduction bit somehow
Rob: No I like that.
I think that that's actually,
that's really close.
That's really close.
So I mentioned right, that a real
core component of our technology
is this interaction between
light and mechanical vibration.
This is a real key ingredient of the
whole process by which we convert photons
between microwave and optical frequencies.
And so light or micro, you know, this
is photonics dealing with photons
and then, the vibrational mode.
These are phonons.
So you have maybe phononics
as the analogous and uh, word.
And really what there was a, at
times in the um, in the community,
they they would look at phoxonics
as the X being a free variable.
Between photon and phonon and
the interaction between the two.
And I think this was really the the
little pun that kind of we built our
name around maybe a little Easter
egg for people who are in the know.
Really?
Dan: Wow.
I feel like I'm in the
know now that's awesome.
Rob: You're welcome.
Dan: Okay, so let's let's go into this
technology a little bit, shall we, first
of all why is Transducing necessary
and challenges does it try to solve?
Rob: Yeah.
Yeah.
So really, transduction is
like a very general term, right?
I suppose if you're trying, you're try,
you're looking at changing something
from one carrier to another, like
a microphone is a transducer, right?
It turns sound waves
into electrical impulses.
And really, so when we say
transduction, it's a, it's a
catch all term for converting.
Quantum information between a
microwave frequency photon and
an optical frequency photon.
So that's the core transduction process
that our technology is is solving.
And what we are really doing there is
we're, so what it, the core reason to
transduce comes down to the different
energy scales of microwave frequency
photons and optical frequency photons.
So you have really interesting and
leading quantum systems, which are based
around microwave frequency radiation.
So say superconducting qubits, right?
They encode their quantum states
in microwave frequency photons,
or quantum dots also interact
with microwave frequency fields,
but the energy is too small.
Such that it can't beat, say,
thermal energy at room temperature.
This is one of the reasons that you
take these systems and you cool them
down in like a dilution refrigerator
to millikelvin temperatures 'cause you
need to freeze out thermal radiation.
But optical frequency photons there a
hundred thousand times higher in energy.
And so you can have single photons well
above thermal noise of room temperature.
So really by performing this transduction
between micro frequency photons and
optical frequencies, what we can do
is we can bring quantum information
that say at the bottom of a dilution
fridge encoded in a quantum computer,
and we can bring that at optical
frequencies out of the cryostat.
And we can now network this
quantum computer with say, other
quantum computers that are in other
cryostats through optical fibers.
And this can be locally like
a local area network in a data
center, but even dreaming bigger.
If you're using telecom wavelength
light, this could be over kilometers,
due to the kind of the propagation
of these optical photons down fibers.
So at the heart of it, what we're doing
is we're allowing by performing this
quantum transduction, we're hoping
to bring quantum information outside
of the cryostat and start connecting
individual quantum processes together
to help them scale into larger systems
and reach the sort of qubit numbers
you need to actually unlock universal
fault-tolerant quantum computation.
Dan: And that, of course is the, is
that's the primary reason why you
would interconnect multiple machines.
Rob: Mm-Hmm
Dan: And we were just talking about this
uh, we talk about this a lot, obviously.
Are there other reasons from
your perspective or, um,
scaling is the thing, right?
And each modality has
its own scaling issues.
And the more people I speak to, the
more I realise every modality is
gonna need networking at some point.
Rob: hmm.
Dan: Keen to hear a bit
more about your perspective.
Rob: Yeah.
I, I think is a really critical
motivator behind developing this
sort of transduction technology.
I think when you talk about every
modality, there's a sort of a larger
dream of transduction, which is, you
know, I think also unlocking the ability
to now combine different modalities
together through these optical photons.
You have people developing optical-only
realizations of quantum computing and.
The and the ability to say, combine the
particular strengths of that sort of
implementation with a microwave frequency
realization like superconducting qubits
or maybe an atomic system, that uses
that communicates with, optical photons.
You could have this interact with
say, a micro frequency system.
So I think that's a in some sense.
I think a longer-term dream
than just pushing, scaling.
But I think this is a really
interesting thing that some that
transduction unlocks is actually being
able to interconnect these different
varieties of qubit definitions.
Yeah and, and also as I mentioned it's
again, longer term, but pushing towards
something like the quantum internet,
being able to combine optical frequency
networking with the computational power
you might get from, say, a microwave
frequency system, like one of these
superconducting qubit-based processes.
I think is a really fascinating
motivation behind this technology.
Steve: So QPhoX is a a spin out from
TU Delft, and I would be curious to
know how is the ecosystem in Delft?
We heard about the UK ecosystem, a couple
episodes back about all these aspects,
but I know that the Delft ecosystem
is, has an advantage, is in the EU.
And I'd be curious to know how did
the, and maybe it's not an advantage,
but it's, you know, UK has the
Brexit and now the next hub in Europe
is in Netherlands, I would say.
So I'd be curious to know
your opinion on that.
How did the Netherlands ecosystem
help QPhoX establish itself?
Rob: yeah.
I think it's, I it's super important, it's
super important to the existence of QPhoX.
Delft is a fantastic place to be
developing these technologies,
as a hub, it is in rude health.
There are, for a long time
now, the university has been
a, an amazing TU Delft, right?
Has been a fantastic center for research
into these quantum technologies.
And now as the commercial field has
emerged, it's very much emerged in Delft.
There are a proper handful of companies
that are all working on really
interesting, complementary problems.
In quantum computation,
.
There's a large amount of um,
collaboration projects across
companies supporting each other.
A lot of us are based now in a building
outside of the university, this House of
Quantum which is a great place, a fully
functioning research and development
center really now for these technologies.
So it's really a fantastic place
to try and get these really
ambitious companies off the ground.
And EU um, EU funding has played
a really important role in getting
a lot of these companies going.
Steve: Yeah.
And I guess the interactions, yeah, of
course it was mostly, yeah, exactly what
I was looking for because I know how
strong it is in the uk, but the EU maybe
is gonna fall behind, but I don't think
so because I see all the activities coming
out of Netherlands and it'd be, it's good
to know that the still holds on some of
that quantum technology with no, I dunno
export licensing and all these problems
so we can collaborate with cross-country.
'cause that, yeah, coming from Germany.
Being able to access some of those
things in the future, perhaps.
Rob: The, the Dutch government I'm a
Brit who's done a lot of work in the
Netherlands and the Dutch government
have made a number of I think really
great tactical choices like the British
government, as well, putting money in
and, yeah, getting involved in really
helping advance these technologies.
Yeah.
Dan: I've got to say House of
Quantum sounds a bit like a
Netflix series, doesn't it?
I'm sure it'd be a great one.
But I guess it's an
accelerator of some kind.
Is it?
It takes some of the pressure
off in launching a startup
Rob: Yeah, I I think, yeah and it's
it's a building, we can join that.
But also, um, we are developing
hardware and this means, especially
developing hardware outside of say,
a university, um, it's not easy.
There's a lot of requirements on
the space, if we can get the right
equipment in the right place with
the necessary infrastructure to get
going, and this is where having these
hub and having other companies you
can pull together to get a building
off the ground is really fantastic.
Not being alone there.
And of course we still work
a lot with the university.
We're independent of course.
But it's, we still plays a really
important role in our development.
Steve: Yeah, that was gonna be towards my
next question, going back into the tech I.
Thinking about, okay, so the
building, the transducer, what
does that actually involve?
What kind of science do you
need to build the transducer?
What does your lab look like?
If you can tell us what's a
typical day in the lab like?
Rob: Yeah, of course, of course.
Our transducers at their core,
it's nanofabricated devices.
So this is work in the cleanroom,
e-beam lithography making the Microwave
and mechanical and optical resonators
that can confine these fields and
enable these conversion processes
that occur within our transducers.
So these are fabricated in
the cleanroom typically.
And of course, it's very important for us
that we develop in QPhoX scalable, where
possible have fabrication techniques.
Each transducer is really small.
It's about a bit over naught
point one millimeter squared.
So you can make hundreds to thousands
of them in a single fabrication run.
And then, but then we're bringing
them out of the clean room and
we're testing them, cooling them
down to millikelvin temperatures.
And then connecting them to qubits.
We have, at QPhoX, we have a number
of really strong collaborations with
people that develop quantum computers.
This is really important to us, right?
We can't we can't uh, silo
ourselves as a company.
We're a connectivity business.
So we, after testing our devices we're now
connecting them to people's systems and
we're looking at the interaction between.
Our transducers and
their quantum computers.
Steve: So I'm trying to visualize what
it would look like to connect transducer
to a superconducting quantum computer.
Is it one transducer per qubit
or is it a single transducer and
then multiplex to the qubits?
Rob: Oh yeah.
So currently, right?
You can connect.
We, we build these systems so they can
be connected in a modular fashion, so
we, they, we can be connected through
coaxial lines and so they can be
multiplexed together, for instance.
Or it could be a one-to-one.
So there, there are quite a few
different connectivity options there.
We don't want the qubit and the
transducer living on the same chip.
That's too close there.
You want to protect and shield your
qubit for many of, say, the optical
frequency radiation, which you
need to have in your transducers.
You want to keep them quite modular.
And apart from one
another to make sure Yeah.
You preserve the quality of your qubits.
Steve: I always thought
it was a transducer right.
In, into the device and then
somehow it manages to produce
the microwaves inside and
Rob: Yeah, exactly.
Exactly.
You can have them all on the same wave
line and the same way you can have
them multiplexed on optical fibres as
well, that then come out of the fridge.
Steve: Interesting.
Dan: so layman's description
qubit, emits, microwave photon.
It's guided into the resonator.
It's somehow interferes with the photon.
Like you must maybe encourage them
in together gently and give them a
bit of TLC, say off you go, and then
they do some kind of interaction
and the photon flies out again.
That's what I'm imagining is
that is, how far away is that
Rob: I I think I, I think you'll, I
think you're pretty close, honestly.
You and you're right to say, you are
right to introduce that photon that
your um, the optical photon, your
microwave photon is intermingling, right?
We, if you want to go between microwave
and optical frequencies there's
a huge energy gap there, right?
You're going from say, five gigahertz
frequency to 200,000 gigahertz
is your how optical frequency.
And you always need to compensate, right?
Energy has to be conserved.
So if you wanna do this conversion,
you always have to send in a laser
pulse, something that can compensate
for that energy difference.
But the rest of it is of
your description Yeah.
Is, is Is pretty accurate.
You, you know, there's
any kind of, there's many.
Preamble, there are many different
ways of doing this, but in, in
this sort of version you're talking
about, yeah, you could emit a photon
from your superconducting qubit.
This could then be, say, entangled
with the states inside your qubit.
And yeah, this micro photon
enters the transducer.
And then now this is in,
in QPhoX' technology.
That Micro-frequency photon gets
converted through piezoelectric
coupling into a micro-frequency phonon.
So a mechanical mode.
And then that mechanical mode is
what interacts with our Optical
laser pulse that we send in.
And then the device will emit a single
optical photon back out onto the
fiber that roots out of the fridge.
Steve: Okay, so then from the
logical perspective, a quantum
algorithm would have to incorporate
some additional stages or let's say
some additional hardware control to
perform additional transduction steps.
It would be, is that a layer
on itself, or how would you see
the transduction in impacting
Rob: Yeah, I,
Steve: deployment?
Rob: no I think that's a really good link
to make, as a lot of quantum computers
right now say Superconducting, quantum
computers just to take a subset, right?
They're based on these, they have
these plaquettes of qubits, and these
qubits are right next to each other.
They're very strongly coupled.
They're very fast interactions,
very noise-free interactions, if
that's the right way of saying it.
Very low noise interaction.
And as you scale up the system,
you look at different forms
of modularity, maybe you have.
Chip-to-chip connections
in the same cryostat.
Maybe they just do, I don't know,
local bonds or maybe they're longer
range, the coaxial cables and maybe
they're ultimately optically realized
interconnects between different fridges.
And I think as you get further
away, yes, the interaction
rate, it's gonna slow down.
Maybe it's not gonna be, maybe
it's gonna have a little more
noise than the local interaction.
And this is something you'll have to
ultimately develop your algorithms
around to compensate for these different
forms of interaction between qubits.
Steve: Okay, so I took a just,
okay, I just realized something
then as you're speaking.
So you just said it, but now it's
clicked in my head what it means.
So the, there's a stage process towards.
Going from multi-chip or perhaps even
single-chip QPU, a quantum process unit.
And then it might be to add
additional connectivity to the chip.
Perhaps you have some qubits on
the bottom right, some qubits
on the top left or something.
And now you need to bring
those qubits together.
Perhaps you can use transduction
there to add additional connectivity.
And then there's, I dunno if
that's even the first step, but
that sounds like a first step.
And then second step would
be multiple chips connected
with the transduction line.
And then to grow that to separate fridges,
Rob: yeah, I think the transducer
really gets involved once you start
looking fridge-to-fridge, because that's
where, why do you need to go to optical
frequencies is because you now want to
bring your signal up to room temperature.
You want to have that sort
of extra connectivity.
Actually, before Dan, you mentioned
other uses of the transducer.
Right.
And I think it is worth kind of, just
briefly mentioning, so this is a, this
single photon conversion is a real
headline application of what we're
developing at QPhoX, but at the heart
of it, we're making very efficient
microwave to optical conversion devices
so efficient that ultimately they can
work down to a single photon level.
And there are actually loads of
really interesting uses for converting
small few photon packets between
microwave and optical frequencies
beyond, say, quantum transduction.
So for instance, if you want to
measure qubits at the bottom of
a fridge, you typically need a
bunch of cryogenic amplifiers.
Like you need an amplifier stage
at four Kelvin in order to have a
sufficient readout signal of your qubits.
That's not that signal isn't
overwhelmed by thermal noise.
You can also, at now the bottom
of the fridge convert your readout
signal, which is not a quantum
signal, it's a classical signal.
Which has say a few photons in it,
you can use a transducer to convert
that into the optical domain and now
measure that at room temperature.
So there's a, a bunch of really
interesting applications of this
technology that's in the few-photon
rather than single-photon domain as well.
. , Steve: what's the timeline?
What's the step-by-step process to go
from zero to connecting quantum computers?
Is it one step?
But that sounds like also very
important use case enhancing measurement
outcomes because those, of course,
getting those corrected is also very
beneficial for quantum computing.
You can measure more accurately
or more simply you can do
better quantum computing.
Mm.
Rob: Exactly.
You can start by saying, okay, how
many, by looking at how I measure
and how I control qubits, how many
can I fit into a single cryo stat?
And you're really looking in detail
at what's the thermal budget I
have of a system that can cool
down to millikelvin temperatures.
And then once you've maxed that
out, you can then also look at,
okay, how do I now start having
multiple systems talk to each other?
And just to keep trying to push
up the qubit numbers, basically.
Dan: Rob, what's the alternative?
Are there people looking at connecting
these dilution refrigerators
through very low temperature
millikelvin connections somehow?
Rob: Yeah.
Yeah.
No, this has been explored.
Yeah, I think the, these are
fantastic tests that people have
done right, where they've built these
superconducting coaxial lines that
connect dilution fridges together.
I think this is an option.
I think this is a challenging option.
Nothing is unchallenging
in, in quantum technology.
I think once you have the transduction,
once you've gone it through that hard
process or relatively hard process of
going from microwave to optical frequency
at the bottom of the cryostat, then
you unlock so much connectivity with
relative ease between these systems
at room temperature, the hard bit is
just get, getting, you get up into
the optical frequency and then you can
get outta the fridge and you can go
metres, even kilometres through the
optical fibre with very little loss.
. So if you're actually really trying
to interconnect lots of systems
together, going through these optical
fibers, having routing and being able
to measure these systems becomes a
lot easier once you're in the optical
domain, which I think is why it's such
an interesting technology for people.
Dan: Yeah.
So you said that's the hardest bit to,
to do the actual conversion transduction.
What are the things that can go wrong?
So what is it sensitive to?
I guess it's heat and vibration,
but then is it different when you
are doing the actual transduction?
Are there, Other things beyond what a
quantum computer is sensitive to that
can affect the transduction quality.
May, you know, do you have to align things
correctly or some other kind of variable.
I.
Rob: Yeah, I think um, so one of
the challenges of the transduction
is that microwave frequency photons
and optical frequency photons don't
couple to each other very strongly.
This is, you can couple them
directly to each other using
non-linear optical materials, but
this is a really weak coupling.
So getting that conversion process
efficient enough such that you can
start converting single photons is
not a trivial problem to solve at all.
And this is why, for instance, we
use these mechanical resonators to
make the system a lot more efficient.
So when you are designing these
systems and building and testing them.
It's all about how efficient is my
process and how noise-free is my process.
But then you also look
at other challenges.
For instance, how much optical power
do I have to put into my cryostat
in order to make this process work?
And is the amount of optical power
I need so much that say I could only
run one transducer in my fridge before
I've heated up the whole system.
So you have to really
look at the whole setup.
Is it gonna be scalable?
How, how many transducers
can you run in parallel?
You also have to say, with these
technologies, you have to look at say
what the bandwidth of the conversion is.
Is my transducer, is it gonna
be compatible with the signals
that come out of my computer?
Can I mode match them well enough?
Such that I have a
really efficient channel.
There are lots of challenges
to get right there.
Dan: I guess there's a bit of a dichotomy
with putting something in that needs
power into the refrigerator that's
cooled down to stop any heat getting in.
So there's a risk is there of doing
too much transduction or perhaps
not being configured in such a
way that it protects the qubits,
the computing qubits themselves.
Like you said, you've gotta
think about the whole system.
Rob: Exactly.
E Exactly.
And this is where a lot of these
challenges in the actual transducer
design and development come around.
Optics and superconductivity
do not mix terribly well.
So there's a lot of hard work in
actually building a design that can
combine these two fields together
and make them interact without them.
Damaging each other at the same time?
Dan: Fascinating.
Honestly, it really is
What I would like to ask actually is
the, was it Piso Electrical effect
that you could, I know it's right at
the core of how the whole thing works.
What is that effect?
And
Rob: Yeah.
Dan: How can you describe it in
words that I could understand?
Rob: Piezoelectricity.
Dan: Yeah.
Rob: Yeah.
I hate to make sure I get
the exact fields right.
Piezoelectricity is the coupling
between, mechanical vibrations and
electrical fields effectively, right?
So this is, say what you have in a
quartz oscillator in your watch, right?
You have a mechanical oscillator,
which is then picked up electrically
and used as a ref, a timing reference
so that your watch stays regular,
Dan: So when you say coupled, the word
coupled means that they're sharing the
vibration, the rate of vibration or their
position, their polarization, their.
Rob: Yeah.
Yeah.
So by coupled it means that,
say a change in electric field.
So if you apply electric field across,
say, a piezoelectric crystal, it
will change shape effectively, right?
So if I apply electric field across
piezoelectric crystal, it will
stretch, ? And then if that electric
field oscillates, it will stretch and
squish and stretch and squish and start
to oscillate itself and vice versa.
That's what I mean by coupled.
So effectively you are transferring
energy between mechanical vibration
and an electromagnetic vibration.
Does that clear it up a little bit?
Dan: A little bit.
Yeah.
Yeah.
On an incredibly nanoscale.
Rob: Yeah, But we are doing
this with very small oscillators.
We are basically taking a piece
of material about, say, 500
nanometers wide, and we are putting
in electric field across it and
making it stretch and squish.
And because it's so small,
the frequency of it stretching
and squishing is very high.
It's, in the few gigahertz this means
that mechanical mode is now at the
same frequency as your the photons
that you are encoding your quantum
information with in your quantum computer.
And so that's how we can take a
gigahertz frequency photon, and.
Translate it into a gigahertz frequency,
phonon, basically a deformation
of this 500 nanometer wide, tiny
block of piezoelectric material on
silicon, which we then turn into we
can then couple to optical fields
and turn into optical radiation.
Dan: actual state is transferred
through the energy transfer,
which is defined in a Hamiltonian
Rob: Yeah.
Yeah, so once you have a, a channel
like this you need, you want to
encode some information into it.
So for instance, you could encode
information into, say the presence
or absence of a photon, and the
presence of absence of a photon will
be trans, A microwave photon, sorry,
would be converted into the presence
or absence of an optical photon.
And as long as you keep everything
low noise enough, you don't corrupt
the information that's encoded there.
And that's what you need
to be really careful.
Dan: Yeah.
Amazing.
Rob: So we, I, we spent a long time
talking about going between microwave
and optical frequencies and then we
took a diversion into Piezoelectricity
'cause we have this mechanical mode.
But it's that use of piezoelectricity
is really important for making
the transduction we do at QPhoX
efficient because one of the
challenges of converting single
photons between such widely different.
Frequencies gigahertz and optical
is that the wavelengths of that
radiation, they're very different.
Gigahertz frequencies have wavelengths
in the, up in the millimeters
and while the optical wavelengths
are down in the single micron.
But by using this piezoelectric coupling
by going from microwave frequency
photon to a microwave frequency phonon,
because the speed of sound is so much
lower than the speed of light, the
wavelength of this phonon, this mechanical
vibration is actually is a lot smaller.
So it's really similar to the
wavelength of the optical light.
And this means that they can
interact really strongly.
Dan: It's like a gearing, isn't it?
Basically, it's, you're like, you're
down gearing the power to allow the
interaction to happen more efficiently.
Rob: Yeah.
You're change, yeah, you're changing
the mode so that it then overlaps.
Yeah.
I think that's a real.
Kind of key component of why we've
of what goes into the design and
the fabrication of our technology.
Dan: Superb.
Thanks.
And I apologize, it's turned into
a bit of a physics lesson, but
Rob: It's starts with the physics, you
Dan: very good.
Yeah.
, what are the unanswered questions?
What don't you know,
Rob: I
Dan: know that you dunno?
Rob: I think, really fascinating process
to take the scientific development and
now bring it out into a company and
actually apply the standards you need in
order to make proper product development.
And I think and I think this is something
the whole field of Quantum Technologies
is going through at the moment, is how
do you actually get the really the the
variability of the devices you're making.
The ex, the exact performance to the
standards where you really actually
have a product at the end of the day.
And I think this is a really interesting
challenge that, companies are developing,
quantum hardware are going through
Dan: Yeah, so not only are
you developing a product.
The fact is they need to go
out into the field, right?
So they need to be reliable, supportable
predictable, those kind of things.
Are you working with, you've
mentioned that a few of your partners
already, quantum computing partners.
How are you preparing yourself
for the different, let's
take Superconductors for a
The number of different
superconducting platforms out there.
Is it a matter of a custom
development for every single partner?
Rob: Yeah, YY yes and no.
Yes and no there.
Like at the end of the day to us,
even if it's a quantum dot or a
superconducting qubit, it's a microwave
photon that we are converting.
But there are specifics between
say, different realizations of
microfluidic qubits and also different
realizations of superconducting qubits.
Say the frequency might
be a bit different.
You know what, some people might
operate at five gigahertz, some
people might operate at six gigahertz
or six and a half, for instance.
And so there is customization there.
But it's, say it's a design-based
tuning of the system.
So it's tweaking the exact design,
but it's not starting from scratch.
There're different quantitative
variations on the same device for
different realizations of qubits.
Steve: Triggered something in
my head when you said standards.
I know it's not the most
interesting topic, but it's an
important topic, especially when
it comes to engineering devices.
I guess it's a, in the
infancy stage, right?
There's no or maybe maybe you
could tell us a little bit what's
the status of standardizing how a.
Microwave-based qubit works to
make sure that your transducers
will always work with the
customers that you want to support.
Perhaps, if you get so much variety,
maybe it's hard to manufacture so many
different varieties of transducers.
I don't know how many there
could possibly be, but
,
Rob: That is such an interesting question
and one that I am, I just personally
say I wish I could answer a bit better.
I know that there is a lot of early stage
work going in at the moment to developing
standards in quantum technology,
starting to lock these things down.
Right now, to the best of my
knowledge, they don't exist.
might check that.
Steve: Yeah, no, I probably, I
would probably agree with that.
I would be
Rob: and so yeah, there is a wide variety.
Again, a lot of these systems are.
Maybe quantitatively different,
but built out of similar
technologies and core ingredients.
And so that may be just
operated at a slightly different
frequency to another one.
Which is one of the reasons why
it's really important that our
technology is modular, right?
And then we don't require
too tight a co-integration.
We make friends with a lot of people
and we work with a lot of people to
make sure that we're up to speed on
exactly how things are operating.
At the same time, I think hopefully, say
you look at dreaming a little bigger,
you look at diverse technologies like
atomic systems or microwave systems,
and you think if you were to define
standards, what would be a great way
to communicate these systems together?
Telecom, wavelength, optical radiation
is a great channel to try and
bring different systems together.
Our devices work in the telecom c-band.
So from 1550 nanometers, that's by design.
We choose to work there because
it's the best place to best place
to scale over long distances.
And I think as we look to developing,
say, networking standards, these are,
these kind of, are great choices.
And then we can, because we can control
basically every feature of our transducer,
it's a design choice as we, we that
we then etch into silicon and build.
We can hopefully be Compatible
with these sort of networking
standards as they emerge.
Steve: Yeah, yeah, especially
everything's still shaping out.
It's hard to put everything on paper and
say, okay, this is how we're gonna do it
from now on when we're so early as well.
So it's probably better if there's no
standard yet as this way gives some
transfer evolution of the technology.
Rob: Yeah.
Yeah.
Yeah.
Dan: And normally when standards start
developing, you get more than one anyway.
So just to mix it up a bit.
It may be that it's in your interest
to stimulate the development
of a standard at some point,
Steve: Yeah.
Dan: In one of the areas to, to suit the
way that your modularity works perhaps.
Rob: Exactly.
Exactly.
Dan: about interactions with
other platforms then, they would
be very different, wouldn't they?
Could you try and guesstimate
for me what a an interface with
a neutral atom would look like?
Rob: I think,
Dan: I.
Rob: That's a great question.
So I it is, like I'm waving the
flag for the optical telecom
band, 1500 Nanometre photons.
Yeah.
I believe in it.
The, one actually, I.
Feature of many atomic systems, right?
Is that they actually they talk to optical
radiation, but they talk to visible or
near-infrared optical radiation, say
rubidium, around seven 18 nanometers.
So if you were to try and talk
to, say, talk from your microwave
frequency system to an atomic system,
you probably need to go through
say, a two-stage conversion process.
You need to bring both
systems to the telecom band.
Luckily, bringing visible wavelength
quantum systems, say trapped ions
or neutral atoms to the telecom
band, is a lot more straightforward.
You're only say halving the energy of your
photons rather than trying to increase
them by a factor of a hundred thousand.
And so this is a, these processes
are a little bit more mature.
But you need to define a common
mode basically through which you
can now exchange information.
And you also need to have this unbroken
channel that allows quantum information
to pass between the two systems.
So you probably need some sort
of nonlinear optical device
to convert between the atomic
system and the telecom band.
But you have, once you've brought them
both to that band, then you can be really
flexible because your light in the optical
fiber, it can go meters, kilometers,
and you can, you don't need to have too
tight a cointegration of the systems.
Dan: So I, yeah, I get the
ability to move up and down
Rob: Yeah.
Dan: wavelengths.
And that there's a much smaller gap
and the no need for a piezoelectric
capability there is there because
it's essentially, it's the photons
are just changing wavelength as
they're going between the systems.
Rob: Yeah.
So converting between visible and
telecom wavelengths, while it's
not completely straightforward,
is a much simpler task because the
wavelength difference is much smaller.
The modes much more readily overlap,
and so it can be made efficient.
It can be made into a very efficient
process, much more straightforwardly
than going from a single micro
photon to a single optical photon.
That's, if you were to start to try and
plug these systems together, that would
be the first challenge to overcome is just
to bring them onto the same wavelength.
Such that they you can encode
information that can pass between them.
A lot of these quantum systems also
have obviously very different dynamics.
Atomic systems are often a
lot slower, for instance, than
superconducting qubits, but they have.
But I think it's a great ability to
be able to actually take advantage
of their different properties.
In, in a computer, a classical
computer, you encode information
in all sorts of different physical
realizations to take advantage
of their different peculiarities.
Why should a quantum computer
have a single physical encoding?
Steve: So we discussed the technology and
the kind of the challenges that need to
be overcome and what's the applications.
Where do you see the technology
going in the next five years?
What do you want to show with it?
What's the, or what's
the big demonstration?
Maybe five years is too long.
Maybe it's too short.
What's the first, what's the
milestone to hit at the moment?
Rob: That's a great question.
So when it comes to actually
this quantum transduction so, um,
converting single photons between.
Microwave and optical frequencies.
I think the big breakthrough that,
you should keep your eye out for
and is definitely something we are
targeting is actually demonstrating now
entanglement between microwave frequency
qubits housed in separate cryostats.
So let's say transferring information
between them, but preparing them
in a highly entangled state.
I think this is a, this would be a really
huge breakthrough that would really
start the ball rolling on connecting
these systems together for QPhoX.
We are pushing towards this, but also as
I mentioned before, we as a commercial
company, we're really interested in
also the earlier applications of this
efficient photon conversion technology.
So there's a lot of work on looking
at, say , performing optical frequency
readout of these qubits in a scalable way.
We just shared last October on the
arXiv results of a pilot study we
did on this with Rigetti computing.
So continuing to develop these slightly
nearer term applications of this efficient
frequency conversion hardware is is
something that we're gonna be really
focusing on over the next few years.
Steve: Yeah, that's something
I'm really interested to see.
Connecting quantum computers, coming from
a networking company, this is, we're what
we're looking at very closely as well.
So, I mean, I'm looking
at it very closely.
See when, you know, then
we need the network layer.
We have the, the ability
to connect for computers.
Now we can start talking networking,
I think, I mean we can already start,
but then it starts being realistic,
.
Rob: I I completely agree.
I, I, I don't want to give the
impression that once you've projected
this into an entangled state.
It's game over.
It's game on, it's the first step
of actually building a networked
quantum data center effectively.
And then of course, you have to think
seriously about, okay, how am I going
to be distributing this entanglement?
What kind of system do I run?
Do I do this on demand?
Am I farming entanglement between
my own computers all the time?
What?
And then of course, Algorithm design.
How do I accommodate the physics and the
rates and the maybe different connectivity
that I can realize between these systems.
Once you have Optical interconnects,
you've got all to all connectivity
between your systems, right?
You have a much more flexible
way of routing signals, and
this really opens up different
alternatives for algorithm design.
Dan: It's gonna be a whole,
another series of trial and
tribulation I guess, aren't there.
And experimentation of
different ways of doing it.
The way you described it there
could be in a data center room,
lots of point-to-point connections
continuous entanglement, distribution
point-to-point, or you mentioned farming.
So I guess that's somehow kind
of building up a collection of
entanglement for consumption there.
There's a whole, yeah.
I'm imagining it's like a,
it's opening the door to all of
this experimentation, isn't it?
Basically, we're waiting
for you, Rob, to open the.
Rob: I'm working on it.
Exactly because like this with any
sort of information transfer protocol
and quantum computing, you can look
at direct transfer, but also you can
also generate entanglement and go
for teleportation based transfer and.
How exactly um, these schemes work.
And also how, for instance, memories.
So lots of people are developing these
optical frequency quantum memories.
How they could enhance the ability
to connect these systems together
is a really interesting question.
Not just relying on the catching,
launching the process and detecting
the photons at the right time, but
maybe using buffering between the
systems I think is a really interesting
option, hardware notwithstanding.
Yeah I it's it's the way that we move
away from this monolithic approach
to hopefully something which is a
lot more flexible and ultimately
scalable when it comes to computing.
I.
Dan: Have you guys got in your
roadmap, some kind of I mean you could
diversify in many different directions.
Um, we've been talking about
different types of transduction.
What about up to the software
layer or the actual networking part
or, where do you see yourselves?
What's interesting you at the moment?
I know it's probably too hard to say
because it's a few years away, but
what's on your mind, what's on your
mind when it comes to diversification?
Rob: I, I, I think that's
a really good question.
At the moment, at QPhoX, our main
focus is all about the hardware,
developing, pushing the performance,
taking it as far as we can, and also.
Pushing partnerships, making sure
that our devices are interacting
with other people's systems and
that we're really demonstrating
the promise of our technology.
I think when it comes to software,
there's the software aspect of
that's of the product development.
That's the software that, the hardware,
I think as a strategy for us at
the moment, it's, there's a lot.
We want to make sure that we're,
we are taking full advantage of our
unique expertise, and that's really
in hardware development and testing.
I think when you are a growing
company, you have to find that
balance between diversification,
de-risking, but also making the most of.
Your unique speciality.
We're the QPhoX, we are the really
first company to really try and
commercialize in a serious way.
I think this quantum transduction
technology, and we want to make
sure that we're really taking
advantage of that position.
So I think that I think there's
a balance with diversification.
Dan: Oh, definitely.
Definitely.
Yeah.
Yeah.
That's why strategy's so important.
Yeah.
Rob: We're thinking
about what we're doing.
Dan: Yeah.
Yeah.
That is, that's a good start.
That's a good start.
Brilliant,
Rob: I'm joking.
But yeah I think that the layer
will be important, but I think
for the moment we have to make
sure that the hardware is there.
Dan: Yeah.
Brilliant.
I just wanted to say thank you very
much for sharing all of that with us.
It's super fascinating to hear what you
are doing down at that level and I'm
very excited to see how your product
development works out and uh, to see
some of the, I'm sure there'll be lots
of public announcements of additional
work with new partners and it'll be
fantastic to see, um, especially that
first shared entanglement between
two different dillution fridges.
Looking forward to that.
Rob: Yeah, I think that that's a, that
would, that's definitely something
to keep your eye out for sure.
No, tha thanks very
much for your questions.
I hope I provided some
illumination on them.
Dan: Yeah, it was good
fun talking to you, Rob.
Thanks very much.
. Bye now.
Cheers.
Steve: Thanks.
Dan: I'd like to take this moment to
thank you for listening to the podcast.
Quantum networking is such a broad domain
especially considering the breadth of
quantum physics and quantum computing all
as an undercurrent easily to get sucked
into So much is still in the research
realm which can make it really tough for
a curious IT guy to know where to start.
So hit subscribe or follow me on your
podcast platform and I'll do my best
to bring you more prevalent topics
in the world of quantum networking.
Spread the word.
It would really help us out.
Creators and Guests
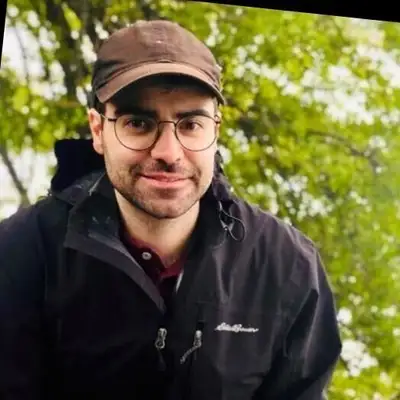
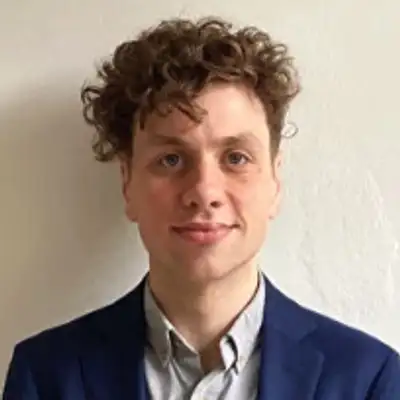
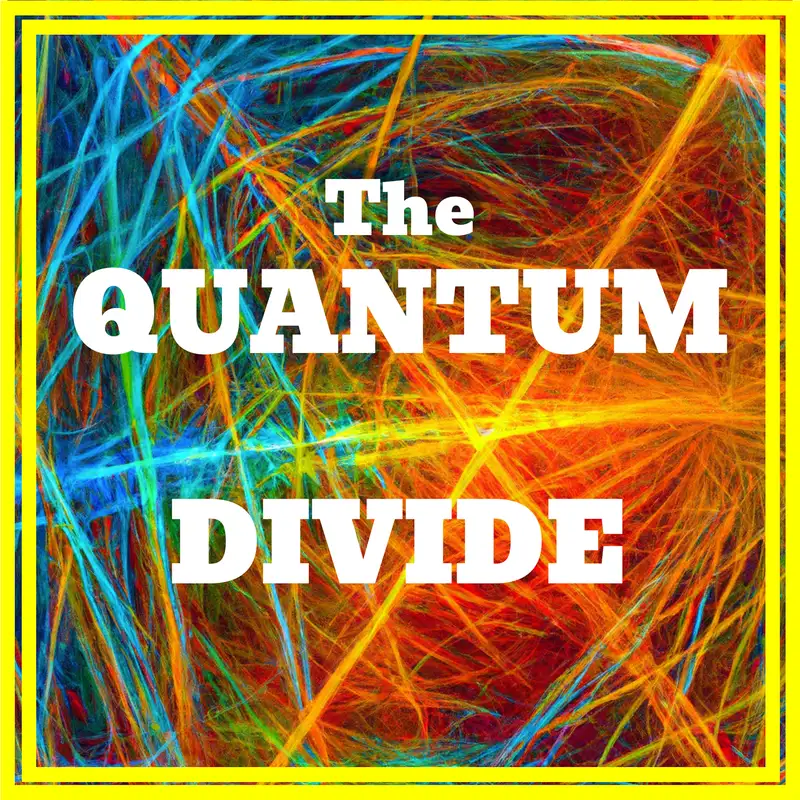