Quantum computing with neutral atoms. An interview with Yuval Boger.
Dan: Hello and welcome
to the quantum divide.
This is the podcast that talks
about the literal divide between
classical IT and quantum technology,
and the fact that these two domains
need to become closer together.
Quantum networking actually is
more futuristic than perhaps
the computing element of it.
But we're going to try
and focus on that domain.
But we're bound to experience many
different tangents both in podcast
topics and conversation as we go on.
Enjoy.
All right, welcome to our next
episode of the Quantum Divide.
And before I introduce our guest this
week, I'm just going to put Steve
on the spot and ask him a question.
It's actually a joke.
Steve, why do quantum information
scientists prefer hamburgers to steaks?
Steve: Good question.
Hamburg is the state, I'm not sure.
Dan: It's because the hamburger
is already in a ground state.
Yeah.
What's the favorite video
game of Schrodinger's Cat?
Steve: I don't know.
Dan: It's Half Life, of course.
All right.
These incredibly awesome dad jokes
with a quantum slant actually
come from QuEra's LinkedIn page.
And the reason why I mentioned
them is we've got Yuval Bogor
with us this week, who is the
chief marketing officer for QuEra.
Welcome, Yuval and and thanks
very much for joining us.
You've got a very interesting background
and as the superposition guy, it's quite
an honor to have you on the call here.
I think we'll start as we normally
do with a bit of a view on your.
Your background your path into
quantum your path into podcasting.
I guess anybody that's listening to this,
that knows the industry has probably
listened to your podcast at some point,
which you're welcome to plug at any point.
Yeah, so keen to hear about your
experience there and and why you do it,
Yuval: It's a pleasure to be here
and not be here at the same time.
Thanks for having me.
So my, my name is Yuval.
I'm chief marketing officer of Quera and
I've my entire life, I've been working
on deep tech startup companies anywhere
from seed stage all the way to NASDAQ.
By education, I have a master's in physics
and an MBA from Northwestern Kellogg
and I've done Many different things.
Enterprise software, wireless power,
virtual reality, agriculture technology.
And then a couple of years ago, I
decided that it's would be a good
time to get into quantum computing.
For a couple of years, I was chief
marketing officer of Classiq, which is
a software company in the quantum space.
And now I've crossed over
to the dark side, to Quera.
We are the leader in neutral
atom quantum computers.
And to your question about podcasts
I found that a fantastic way.
This is not my first podcast.
I'm doing the superposition
guy podcast these days.
I used to do the qubit guys podcast.
The classic, but even in other
industries, I was the VR guy when doing
virtual reality and the charge guy
when doing wireless charging and so on.
It's a fantastic way for me to
both learn talking with all these
smart people, and it's also been a
fantastic business development tool.
Once the microphone is off.
You can talk about other things beyond the
podcast, and it's a, it was a very good
way for me to network into the industry.
Steve: We were curious about
the different names you had.
Super position guy, qubit guy, charge guy.
We're thinking what's now it's clear why
there's so many different names because
I guess every time you switch positions,
you have a new name for your podcast.
That's great.
Yuval: Absolutely, and that, that
used to be the the litmus test
for, is an industry young enough?
Because in virtual reality, for
instance, when I saw that VR
guy was available, I said, okay,
this is a young enough industry.
And when the qubit guy was
available, I said, oh, quantum is
a young enough industry as well.
And so this fits what I love to do.
Steve: yeah, that's great.
So I think, when it comes to
the quantum part of the quantum
podcast, you've had many episodes.
So one thing we're curious about is
there something you noticed across these
episodes that's something in common or
something vastly different between them?
What kind of trends have you
observed with the people who work
in quantum that you've interviewed?
Yuval: I think you have to be
an optimist to work in quantum.
Because the dark secret is that quantum
computers are almost useless today.
Other than a few esoteric applications.
There's nothing you can do on a quantum
computer that you cannot do classically.
So you have to be an optimist
saying yeah, that's right.
It's now, but in two years or three
years and five years, just wait
and see all these amazing things.
And it does make sense.
You got to walk before you
run and crawl before you walk.
So we're somewhere between crawling
and walking in quantum, right?
So I think most of my guests
have been very optimistic about
where the industry is going.
Otherwise they probably wouldn't be here.
Steve: Yeah, it makes sense.
It's a, in this position of
being risky, but promising.
And I can see that perspective.
You need to be optimistic.
You have to believe in hard
problems can be solved,
okay.
Yeah.
So then again, towards the trends of
the podcast, I guess it's, since you
started, things have maybe have changed
with perspectives and what people are
focused on and what people believe in.
Have you noticed a shift in, for example,
what people focus on the research or
have companies pivoted since you first
interviewed them and now they're changed?
Have you noticed something changing
in the industry throughout the
time of interviewing your guests?
Yuval: Absolutely.
So the industry does
move in a fantastic pace.
The number of qubits that are available
out there, the variety and the modality
of the machines is something that was
unimaginable three or five years ago.
One thing that I found surprising
but is a very sort of prominent
thing that's happening is the move
towards on premises quantum computers.
If you ask people three years
ago, would someone need an
on premises quantum computer?
I think the answer would have
been almost universally no.
And the reason is quantum
computing moves very quickly.
Some people say by the time I buy
and get delivered quantum computer,
is it going to be obsolete?
What's the useful life?
of the quantum computer, a cloud lets
me experiment with all these quantum
modalities relatively inexpensively.
So there are a lot of things going
for cloud based quantum computing, but
then again, people want on premises.
So we started exploring
why that's the case.
One reason is the emergence
of national quantum programs.
So a lot of countries around the world
are starting in country quantum programs.
Whether because they believe it's the
next big thing and some of these countries
feel that maybe they've missed the
Internet or maybe they've missed the A.
I.
Wave and they don't want to miss quantum.
So they're investing millions and
sometimes billions of dollars in
creating a local quantum ecosystem.
And for them, having quantum computers.
In country is an important seed
in creating that ecosystem.
If I'm in Denmark, then it's
probably not good enough for me to
have a quantum computer in Boston,
Massachusetts, where QuEra is.
I want it in Denmark.
I want it in country.
So that's one reason that's happening.
The other reason that's happening is as
quantum computers become more useful,
people want more control over who uses the
computer, how soon, and the priorities.
Because if you go to a cloud service, you
may be hours or sometimes days behind some
other large jobs that are in the queue.
But if quantum is becoming
mission critical for you, then
you say no, I want to run my job
now and I'll set the priorities.
And oh, by the way, I want the computer
to run 20 hours a day and not seven.
And so having that control sometimes
leads people to the on premises.
And then there's always the defense
contractors or other people that feel like
they need an air gap system that they just
don't want to send their code over the
internet and want an on premises for that.
So that's been a major shift that we've
observed over the last six to 12 months.
Dan: thanks Yuval and I guess sovereignty
is very important where in a macro
environment where globalization is
diminishing a little bit and all of
those kinds of drivers supporting that
That paradigm you've just described.
I guess let's go on to
your day job, shall we?
So give us a bit of info about Q Uera.
I understand it's a neutral
atom architecture, but I guess I
mentioned to know about the company.
Why neutral atom?
And yeah, we can go on to have some
more technical questions after that.
Yuval: Absolutely.
So there are various modalities,
various methods of building
superconducting qubits.
Some people may use photonics or others
use trapped ions and so on and we use
neutral atoms So neutral atoms in our
case, we use rubidium 87 So rubidium is
on the left side of the periodic table
a couple rows a few rows below hydrogen
and Rubidium is very interesting.
I mean in general when you
when your cubits are atoms
there's no shortage of them.
You can make as many as you want.
They're all identical.
There are no manufacturing
defects or inconsistencies.
They're very small
because they're neutral.
They're less susceptible to
environmental charges or other things
that are happening around them.
So Quera started about four years ago.
It's an outgrowth of amazing work
that was done at Harvard and MIT.
Harvard and MIT built.
Many years ago, the first I think
at the time, was the largest
computer in the world, 51 qubits.
And then Quera was started
to commercialize this, and of
course, add our own discoveries.
We believe neutral atoms are, is
the right approach, of course,
otherwise we wouldn't be doing it.
We think that neutral atoms are scalable,
meaning as you go to larger and larger
number of qubits, other modalities
require optical interconnects or other
sort of ways to distribute the qubits.
And with Quera, we believe that we
can get to at least 10, 000 qubits
without needing an interconnect.
And in fact, As we speak, and
actually since November of 22, we've
got the largest publicly accessible
computer, a 256 qubit machine
that's available on Amazon Bracket.
We're the only Neutral Atom vendor that
has a computer that's publicly accessible.
Part of that is due to the ingenuity
of the Quera scientists, and part of
that because of the amazing work that
was done and continues to be done at
MIT and Harvard and our collaborators.
Another advantage of neutral atoms
is that you can move them around.
Basically, we can get into how we do
computations, but the atoms themselves
are suspended by thin laser beams.
They're suspended about
four microns apart.
That's four millions of a meter.
Sort of science fiction at its best.
And by moving the laser beams,
you can move the atoms around.
And what that means...
is that you can have what we
call any to any connectivity.
You can get any qubit to interact with
any qubit, any other qubit directly.
Now, why is this important?
Imagine that I'm at a cocktail
party and you see me next to the
punch mo punch bowl, my usual spot.
And I spot someone at the other
side of the room and I want
to go to speak to that person.
So I just go over and we chat
and we exchange information.
Now, if I was glued to the floor, and
I wanted to speak with someone at the
other side of the room, I would have
to pass the message to the person next
to me, and then next to that person,
and so on, and maybe play telegraph,
and maybe 12 people down, then some
message would get to the other side.
It would take more time, it's
much more prone to errors.
That's the difference between neutral
atoms, where you can move the qubits
around to talk with any other qubits
and say superconducting, where the
connectivity is fixed, where every qubit
can speak with, say, four other qubits.
So we can create more efficient programs
and we can create some amazing things
that we believe are not possible or at
least very hard with other modalities.
Dan: Excellent.
Thanks.
So my understanding is the Dead.
The atoms of rubidium
are suspended as a gas.
Is that right?
And the lasers create a lattice
of some kind of these atoms.
And then that's what you perform
the computation on top of.
Is that a good description?
Yuval: Yeah we take rubidium
atoms, we trap them in these laser
beams, The laser beams sounds
counterintuitive, but it's true.
The laser beams cool down the atoms
to very close to absolute zero.
When people, lay people think about
laser beams hitting stuff, that's
usually about, oh, they're heating it up.
They're actually going to make it warmer.
In this case, there's a direct
relationship between the temperature
and how quickly an atom moves.
So when an atom is trapped, it's
essentially considered that.
Very low temperature.
And the way these work, what we call
laser tweezers or optical tweezers,
is when an atom starts moving in
the wrong direction, the photons
in the laser actually push it back.
The laser is tuned to push it back.
It's almost like the Doppler effect.
And so the laser beams are able
to trap the atoms in place.
And then this array of laser
beam, one for each qubit, We can
arrange them in different ways.
Now, today, we use two
energy levels for each atom.
We can think about them as zero and
one, or sometimes they're called
the ground state, back to your joke
from earlier, and the Rydberg state.
A Rydberg state is a state where
one of the electrons in the
rubidium atom is excited to a
high energy high energy state.
And when excited, that atom appears
to be thousands of times larger
than when it is in the ground
state, in the lower energy state.
And the reason that's important is
if you take two atoms, if they're far
apart, they can both be at zero or
one, both be ground and Rydberg and
they don't interact with each other.
But if you get them close enough,
then there's this thing that kicks
in called the Rydberg blockade.
And the Rydberg blockade prevents
one atom to be in the Rydberg
state when the other one is.
So all of a sudden, you have a
situation where 0, 0 is allowed,
and 0, 1 is allowed, and 1,
0 is allowed, but not 1, 1.
So that's the simplistic
explanation of how you could do
a XOR gate with Rydberg atoms.
So by arranging the atoms around, So
that we know which atoms, which qubits
are interacting with which and then
exciting them, moving them up to the
Rydberg state in some fashion, we can
create computations, both what we call
analog mode computations, as well as
what people usually refer to as universal
digital gate based computations.
Dan: It's fascinating and
quite sci fi, as you described.
Neutral atoms, they've really,
from what I can tell, come on
accelerated in their kind of purpose.
In relative years I only just listened
to another podcast and I don't mind
pimping somebody else's podcast on
mine, but the new quantum era with
Sebastian Hassinger and Kevin Rowney,
they just interviewed the chief strategy
officer of infleqtion, and he was
talking about neutral atoms for sensing
primarily the sensors to, to measure
changes in the environment and so on.
And I guess the big benefit is that
they can run at room temperature.
The, obviously the applications and
use cases are significantly broader.
Is it, do your computers run
at room temperature as well?
Is there a fridge inside
inside your systems?
Yuval: They absolutely
run at room temperature.
And by the way, the previous podcast
that Sebastian Haslinger did was
with Alex Kiesling, the CEO of Quera.
So he was talking about
neutral atoms, of course
Dan: Fantastic.
There you go.
Yuval: So yes, we run at room temperature.
We do not need cryogenic.
Coolers, which is sometimes amazing.
On one hand, it deprives us from the
sort of iconic look of the quantum
computer, the golden chandelier.
But on the other hand, fact that we don't
need cryogenic cooling means that it's
easier to scale and it's also easier
to deploy when we go and look at HPC
at high performance computing centers.
And so do we need a big room
for your fridge and so on?
So no, you actually don't.
Our computer today.
It takes about four 19 inch racks, consume
about, consumes about seven kilowatts of
power, that's three hairdryers doesn't
require any special cooling just requires
sort of normal HPC room temperature in
our air condition, no condensing humidity,
the thing you'd expect electronic
equipment to work in and that's it.
So it's really simple.
To deploy and of course, we save a
lot of money by not needing a dilution
fridge and save a host of other issues.
Dan: Yeah.
My understanding is that the
technology in the dilution fridges
is it's come on so well that they're
actually very easy to implement, but
you still have to have one, right?
And in that case you are cutting
back the applicability of the
technology in certain domains.
Just one more thing on neutral atoms.
Are there any unique error correction
techniques which are used in when you have
a lattice of atoms controlled by lasers?
I guess it's a very Delicate process and
balance that has to sit in the system.
Yuval: Neutral atom error correction is
actually, of course, it's very important.
That's sort of part of the path of the
future to truly usable quantum computers.
And we believe that neutral atoms
have some unique advantages.
One thing that with an error
correction code, you usually want
to spread it over a larger distance.
And so the fact that you can move
atoms around is great because you can
say I'm going to entangle them, I'm
going to encode them and then spread
them around as I want geometrically.
The other thing, if you can imagine
a logical qubit an error corrected
qubit that's made of a couple dozen or
a couple hundred of physical qubits,
you can move them around, each logical
qubit, you can move them simultaneously,
and then perform operations on all
the physical qubits at the same time.
So that's also great.
The other thing, just recently there was a
paper published by Harvard and Quera and a
couple of other institutions showing that
the ability to move qubits around leads
to more efficient error correction codes.
So this is QLDPC and they
basically said by moving the atoms
around, we need fewer qubits.
And that's a big deal because if you have
a system with 5, 000 qubits, and you need
a 500 to 1 physical to logical ratio, then
from 5, 000 qubits you get to 10 qubits.
However, if you only need, say, 50
qubits, you get to 100 logical qubits
with the same number of physical qubits.
These efficient error correction
codes are truly important.
And we believe they're a key factor
key advantage of neutral atoms.
Related to that, think about it this way.
When you think about classical
computer architecture, you have a
memory, and you have a CPU, where you
have registers that you can perform
operations on, and then input and output.
By moving qubits around, we
can create something similar
in a quantum architecture.
So imagine that we had three zones.
We had a memory zone where the
qubits have a very long lifetime,
perhaps multiple seconds.
We have a processing zone where we
can bring qubits from the memory
zone, perform operations on them.
And then we have a measurement zone,
which allows us to do mid circuit
measurement, which is important for error
correction and other things as well.
The fact that you can arrange a processing
zone that's separate from the other
zone also helps with scalability.
Because today...
In a superconducting architecture, for
instance, I believe that every qubit
gets three wires, three control signals.
So if you need 10, 000 qubits,
you're going to have 30, 000 control
signals, three signals for each qubit.
But if you have an architecture
where you just move...
qubits into an area where you do
processing and then control them, then
you don't need to scale the same way.
You can scale much easier
with much fewer controls.
Think about the absurdity
of, for instance, if you
opened your 4K television.
And you found three wires
going to every pixel.
That's going to be a lot of wires
and that's going to be a very bulky
TV if it could be made at all.
So the ability to multiplex, the
ability to scale up the number of
qubits without significantly scaling
up the control signals is another
key advantage of neutral atoms.
Steve: So we talked a lot about the
advantages of the neutral atoms.
Would you say there's any specific
disadvantage that is based only
neutral atom to architecture?
Yuval: Of course no technology is perfect.
So one disadvantage of
neutral atoms is speed.
And what I mean by that is for a neutral
atom computation cycle to happen, what,
what is basically happening you get all
these rubidium atoms, you arrange them
in any way you want, you do the actual
operation, you excite them, and then
you take a photograph or a video of them
with a camera and you process the image.
Now, the middle part, the execution,
is really just a few microseconds.
But setting up and then image
acquisition and image processing
could take milliseconds.
And therefore, neutral atom quantum
computers might be limited today
to just a few cycles per second.
I'm sure it will improve in the
future, but it's not going to
improve a thousandfold in the future.
Whereas superconducting qubits
can do many more shots per second.
So if you've got an operation that
needs millions and millions of shots,
Then from a shot, from an execution
type perspective, you're probably
better off using some other technology.
Now, it may be more noisy, you may or
may not get the results you want, but
at least it's going to operate faster.
So that's one disadvantage
of neutral atoms.
Steve: Okay.
Yeah, very interesting.
So a little bit of a deviation here.
So we briefly touched on high performance
computing, which leads to the question
of, okay, we know some applications of
quantum computing could be in quantum
simulation and quantum chemistry.
But are there any applications you
see that kind of merge HPC and quantum
computing that are maybe inside
the scope or outside the scope that
maybe aren't as popular as those?
Yuval: I, I think it's, you mentioned, we
were talking about trends in the market,
and I think more and more people realize
that it's unlikely that the quantum
computer will be a standalone quantum
computer not connected to any Classical
CPU or a GPU or a graphic processing unit.
There are many reasons for that.
One is just input output.
Quantum computers are not very good in IO.
It's unlikely that we would ever
run zoom on a quantum computer.
So you certainly need classical part.
But then, unless you're a purist, you're
basically saying, Okay, I've got a
CPU, I've got a GPU and I've got a QPU.
Let's divide up the algorithm
in a way that each machine
does what it's doing best.
So a GPU maybe is going to run
on the machine learning tasks
or certainly image processing.
A classical CPU may do input
output or, again, Microsoft Word.
I don't think it runs on a GPU today.
It doesn't need to.
In the QPU, we do something else.
So we see a lot of
these hybrid algorithms.
That basically cycle back between a
quantum portion that does something
and then a classical portion that
measures the results, adjusts some
parameters, and takes it back.
So I think there'll be, I would
venture a guess that most algorithms
would not be pure quantum algorithms,
but they would run on some
combination of classical and quantum.
Steve: Do you see that as the way
that quantum computers will make the
biggest impact and will be able to
scale with the size of the problem?
Or how do you see that?
Yuval: I don't know if it's so
much the way to scaling, but
rather the way to usefulness.
People have built data centers.
They've got lots of compute power there.
They've got lots of storage
where they could store the data.
Now, if they want to take part of the
data and process it using a quantum
computer it's gonna end up being a hybrid.
If they want to communicate that
it's likely going to be classical.
If they want to build off work
GPUs, that's gonna remain there.
So we see it as a path to usefulness.
And indeed when we speak with HPC
centers today, a lot of them come up
and say we on, we want your quantum
computer to be HPC ready, which means
it could interface with classical
computers, which mean that even from
a management perspective, security.
Authorization, billing, job
scheduling, synchronization,
orchestration, all of these things.
They've are understood today
in the classical world.
We don't have to reinvent the wheel.
We want the quantum computer to fit into
that kind of existing infrastructure.
Steve: And do you think that It
will be necessary to connect those
quantum computers via quantum
network or will it only require
classical interfacing between them?
Yuval: I think that in many cases,
classical interfacing will be sufficient.
And actually When we spoke about error
correction and about camera readouts
and all these things, sometimes
it can be very useful to have a
GPU that's really tightly coupled.
Very close, microsecond latency with
the quantum computer, not because it
needs to run some hybrid algorithm,
but because that GPU can help shape
the pulses or optimize the quantum
computer or help with error correction
or help with image processing.
And so we're actually seeing
classical processors come closer
and closer to the quantum core.
Quantum networking might
be useful if you want.
Distributed quantum computing
but in a data center that's co
located, I think that might not
be, that might be an overkill.
Dan: So I get the feeling then that
perhaps distributed quantum computing is
not something that QuEra is looking at.
Is that right?
That you're keeping all of the
compute power within one system.
Because you, perhaps it's an easier system
to scale than maybe a broad, Statement
that you're welcome to shoot down.
But that lattice, essentially, you're
planning to grow that rather than
look at distributed quantum computing.
And at some point
Yuval: we're just early in the market.
When you look at a classical supercomputer
that's made of hundreds or sometimes
thousands of CPUs and GPUs, but each
of them is powerful in its own right.
So right now we're in the process of
let's develop that individual unit before
we start connecting them all together.
The distributed quantum computer.
Can certainly, you can think about it
as oh, I've got five quantum computers
in different cities, and if I could
network them and get them to run some
coherent algorithm, then maybe I could
exponentially increase the power.
But we're just not there yet
in terms of we just need to
develop the individual Lego block
before we build the full castle.
Dan: Great.
Yeah.
One step at a time, Dan.
Slow down now.
Okay.
I actually had one more question.
I'm sorry.
It's taking a step back.
But when we were talking about the the
lasers or essentially the photonic beams,
if you like, which are holding the atoms
in place, is it those same lasers which
carry the signal to, as you put it, excite
the atoms and position them in such a
way that the computation can happen?
Or is it happening with Separate lasers.
Yuval: So you've got
multiple things going on.
You've got lasers that
hold the atoms in place.
You've got lasers or other,
electromagnetic signals that can
move the atom from the ground
state to the excited state.
You have other signals that cause them
to interact this way or the other.
So it's a complex system.
It's not just one wavelength or
it's not just one excitation.
Dan: Thanks.
And hey, I'm the IT guy on this call,
so I get to ask the stupid questions.
Yuval: I'm the marketing guy.
I get to answer the stupid answers, right?
Dan: Yeah.
We make a good team then, Yuval.
So you mentioned logical
and physical qubits.
I don't have that straight
in my head on how that works.
How does the mapping work
inside your system for that?
Is there a set of mathematics
which results in the way the
qubits are interoperating?
That you send a signal to multiple
qubits and you get the result from one?
Or, how 10 qubits provide the
function of one logical qubit?
Yuval: That's an excellent question.
And in fact, I just published or the
Quantum Insider was kind enough to publish
a pretty long article that I wrote about
error correction and why it's needed and
what the state of the art is and so on.
But, let's start from the beginning.
Error correction is needed because
qubits are fragile and even today,
the state of the art for two qubit
operations, taking two qubits and doing
something with them is, let's say, 99.
8%.
That sounds like a lot.
But it basically means that two out
of, two times out of a thousand,
the result would be incorrect.
So if you've got an operation that
has a couple of hundred of these two
qubit operations sequentially, then
you're more likely to get junk at
the end of that than a true result.
In contrast, a classical computer, the
error rate may be 1 over 10 to the
power of 20 or something like that.
Orders and orders of magnitude different.
Logical qubits are, is some scheme
to basically say, let's take multiple
physical qubits and see if we can create
a qubit that's resistant to error.
That I, we could detect
and correct errors.
Now, if we were doing this classically,
so if we were recording, I needed to
give you my credit card number and we
were on a really noisy phone line, maybe
I would repeat every digit a few times.
So if the, if my credit card
starts by 4162, maybe I say
444 111 or 444 666 111 222.
And I sent you every digit three times.
So hopefully, if one of them is
misheard, you can say, Oh, yeah
I know how to detect that there
was an error here and correct it.
And if the line was even more noisy,
maybe I do every one five times or
nine times or something like that.
So at some point, depending on how
resistant I want the code to be to
noise, I can repeat the number of digits.
And of course, classically,
you can do smarter things.
Unfortunately, in quantum computing,
you cannot replicate a qubit.
There's a law of physics that's
called the no cloning theorem.
You cannot take a qubit
and just make copies of it.
That's, it's a double edged sword.
On one hand, oh, it's really bad, I
wish I could, because that, then, for
error correction, that would be great.
On the other hand, when people talk
about secure quantum communications,
then the fact that you cannot clone
a qubit leads to that resistance
to tapping, to wiretapping, right?
Because if someone were to listen
in and try to copy the qubit
that's running on the link...
I would know, I would find out, so
that sort of gives the resistance.
Quantum computing had to come up with
different codes on how to encode qubits.
And the size of that code, the
number of physical qubits that
make up one logical qubit, depends
again on how resistant you want...
that to be to errors.
So the people talk about
what's called code distances.
How many errors can
you detect and correct?
And the larger the code distance,
the more physical qubits you
need for a logical qubit.
Now imagine very simplistically.
That if a logical qubit had five physical
qubits, I wish that was the case, but
that's usually a higher number, then I
could take these five physical qubits,
perform an operation on these five
pairs, one qubit from each logical qubit,
and then see what happens, correct the
errors, and move on to the on and on.
So that's basically what
people are trying to do.
They're looking at different codes, how
do I encode information in a way that's
resistant to errors that I can correct it.
How do I do that efficiently?
But the ultimate goal is to reach a
situation where, yeah, there's a lot
of stuff going on underneath, but
at the end of the day, you trust the
calculations and you can do sophisticated
calculations with many operations
without worrying about quantum errors.
Dan: Thank you for that detail.
It's the way I see it now then is that
it's because it's a probabilistic system.
It's not deterministic
like a classical computer.
So if you have lots of errors,
you're going to get that's going to
affect your end probabilistic state.
So the more qubits you can perform
the Calculations on then the higher
chances that the probabilistic
state is going to be more accurate,
Yuval: that's one way to look at it.
But yes, you're basically trying to create
a mechanism to create and correct errors.
Otherwise...
You're not going to get to
useful quantum computing.
Dan: right.
Okay, and I've got the first few digits.
If you could just give me the
rest of your credit card number,
that would be good as well.
Yuval: Absolutely.
Dan: And of course, I'll put a link into
the into the show notes for your article.
I'm happy to do that.
Yuval: And the credit card, right?
So because it's
Dan: No, I'll keep that to myself.
That's fine.
Steve: All right.
Then the next topics are more on the,
we talked about the hardware, talked
about the error correction, talked
about how to perform quantum computing.
And now the question of course,
is what's going to be the thing
we do with the quantum computers.
What's the application
of the quantum computers.
So one question is what's the application
you think is the most promising right now?
And so one thing I'm curious about
is particularly quantum machine
learning, where do you see the
application that will make the first
impact, let's say, maybe not the
biggest impact, but the first impact.
Yuval: Today we see three classes
of applications that people
run on our quantum computer.
Simulation, optimization,
and machine learning.
So simulation, that goes back to
Richard Feynman that basically said the
best way to simulate a quantum system
is to run it on a quantum computer.
So if you have a system with quantum
mechanical effects, then it's
sometimes much easier to experiment
on a quantum computer doing that.
So even with our system today, we
think that 256 qubits is a lot.
Today in 2023.
But, in 10 years, it will be nothing.
It's going to be a four K ram equivalent.
But we are seeing scientists that
are able to simulate and measure
effects that were previously predicted
theoretically, but not observed.
So sort of unique states of matter,
for instance, or things, for instance,
there's a topic called spin liquids.
And so a lot of amazing things
that people can simulate on our
existing computer, the second topic
is optimization and optimization
is really a universal problem.
Everyone wants to
optimize something, right?
FedEx wants to optimize.
The routes of their drivers Fidelity
wants to optimize the portfolio, a
telecom wants to optimize the placement
of the 5G cell towers, and on.
So we see a lot of optimization work, and
one of the interesting things about the
way our quantum computer works is that
We spoke about the ability to move qubits
and to place qubits in various locations.
We can encode some of the constraints
in optimization by moving the
qubits to the right place, by
the geometry of the qubits.
So every optimization, or most
optimizations, have constraints, right?
I want to optimize the portfolio.
But I'm constrained in how much money
I can spend on the stocks, right?
I have to the value of the stocks
needs to be, I don't know, 1, 000.
So one example that we show on our
website is a store placement example, or
you can think about it as a let's say an
example of ATM cash withdrawal machines.
So you're a bank, you want to place ATMs.
You want to place them in areas that
people want to take out cash, but you
don't want to place two ATMs super close
to each other because then you're serving
the same client base and you just put two
machines, whereas one would be enough.
So we've solved a couple of these
problems for our customers and they
basically say here are all these...
Candidate locations that we can
put the ATMs or put the stores.
Here are the constraints that we
don't want these and these stores
to be too close to each other.
And then we run it through the
quantum computer and through that
Rydberg blockade mechanism, the fact
that the system does not allow two
atoms to be in the high state and
the Rydberg state simultaneously.
Think about it as I cannot place
two stores in these two locations.
We can solve a lot of
optimization problems.
Perhaps better than other machines.
And the third area, as you mentioned,
is quantum machine learning.
And quantum machine learning
usually has two parts.
One is classification, right?
I've got images, dog or cat,
or, you could certainly do
something much more sophisticated.
Or you have prediction where you say
this was the water consumption in a
certain city over the last two weeks.
And what do we expect the water
consumption to be tomorrow at 8 a.
m.?
So take historical data and
extrapolate it into the future.
Initial attempts at this were to
take classical algorithms and just...
Run them on quantum.
And that's proven to be very
difficult because quantum computers
are noisy, meaning they're not
precise in their measurement.
So either you have to run many
measurements, or you get results
that are not fully accurate.
And so the process of teaching a network,
of training it, was very difficult when
you used classical algorithms and tried to
just implement them on quantum computers.
Fortunately, there are other approaches.
One of them that we like is called
quantum reservoir computing.
And reservoir computing is pretty cool.
It's basically saying I've got inputs, I
encode them somehow, and I then use these
inputs to excite a reservoir of something.
Think about it as I can drop stones into a
pond, and let's say the location of where
I drop the stones is relating to my input.
And then I measure ripples on
the pond in some other location.
And the pond, because this body
of water where they got all these
interactions going on that I may
or may not understand creates some
connection between the input, what I,
the stones that I threw, and the output.
And it turns out that you can do this with
a quantum system, run training through
it, look at the outputs, use classical
training to match the outputs of the pond
with what you're trying to determine.
And you're actually
getting very good results.
We were getting that in smart factories.
We're getting that in
handwritten digit recognition.
We're getting that in extrapolating
time series to the future.
So there have been some really exciting
work that's happening in quantum machine
learning not running chat GPT on quantum
anytime soon, quat GPT, but a different
set of problems that are showing promise.
Steve: So you think the direction
of taking the classical input.
Converting it to a quantum state
or encoding it into quantum states.
This will be too challenging for the
near future, or at least maybe forever.
What do you think?
Yuval: I think that quantum brings
new capabilities and you have to
think about problems differently.
If you just try to take a problem that you
solved one way and force feed it into a
new type of computer, just like when you
started using GPUs, you could think about
parallel processing and you can think
about what each pixel or each core can do
and not just think about a program that
runs sequentially like a classical CPU.
So once you started thinking
in parallel for GPUs, you
could do some amazing things.
Once you start thinking about
superposition and entanglement and
interference in quantum, that's where some
really cool stuff is going to come out.
Dan: It's a totally
new paradigm, isn't it?
And that's the thing when new paradigms
come about, you don't really know what
the final kind of value is that you're
going to get from different use cases.
Of course, we see a lot of them and
you've covered a lot on this call.
So thank you for that.
It's a really interesting,
broad discussion.
And I think probably a
good time to to wrap up.
Yuval so listen.
Thanks so much for talking to us.
Is there anything else you'd
like to close it off with any
closing comments or thoughts?
Yuval: we're in the
beginning of a journey.
It's a truly exciting one.
And there's room for everyone if you're
a physicist, if you're a computer
scientist, if you're an engineer,
this is like to take prototypes
and bring them to production.
So it's not handcrafted quantum
computers, but production line.
If you're thinking about if you're
a computer science major that wants
to design new algorithms to take
advantage of these amazing capabilities.
Or maybe if you're just a technician
that's going to keep the machine
running and at peak performance,
there's room for everyone.
It's an industry that's very open
and friendly, and I encourage
everyone to get involved.
And of course we believe in neutral atoms.
So check us out at QuEra.
com.
But whichever modality you choose
we, we hope to see you in the
Hilbert space and the quantum space.
Very soon.
Dan: Of course.
And you don't need a quantum
background to go into quantum.
I think that's the other thing, right?
Of course, when you finished listening
to this episode perhaps now you could go
look on your podcast client and look for
the super position guy and hit subscribe.
I'm sure Yuval would love that too.
Yuval: Absolutely.
Dan: Thanks very much.
Yuval: Thank you guys.
Steve: Thanks
Dan: I'd like to take this moment to
thank you for listening to the podcast.
Quantum networking is such a broad domain
especially considering the breadth of
quantum physics and quantum computing all
as an undercurrent easily to get sucked
into So much is still in the research
realm which can make it really tough for
a curious IT guy to know where to start.
So hit subscribe or follow me on your
podcast platform and I'll do my best
to bring you more prevalent topics
in the world of quantum networking.
Spread the word.
It would really help us out.
Creators and Guests
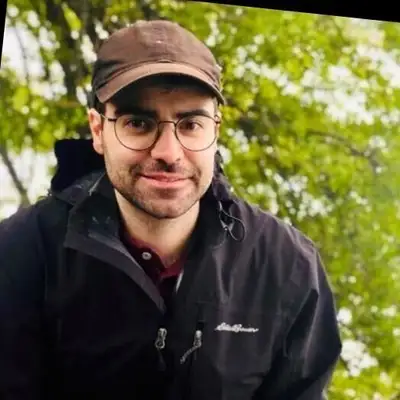
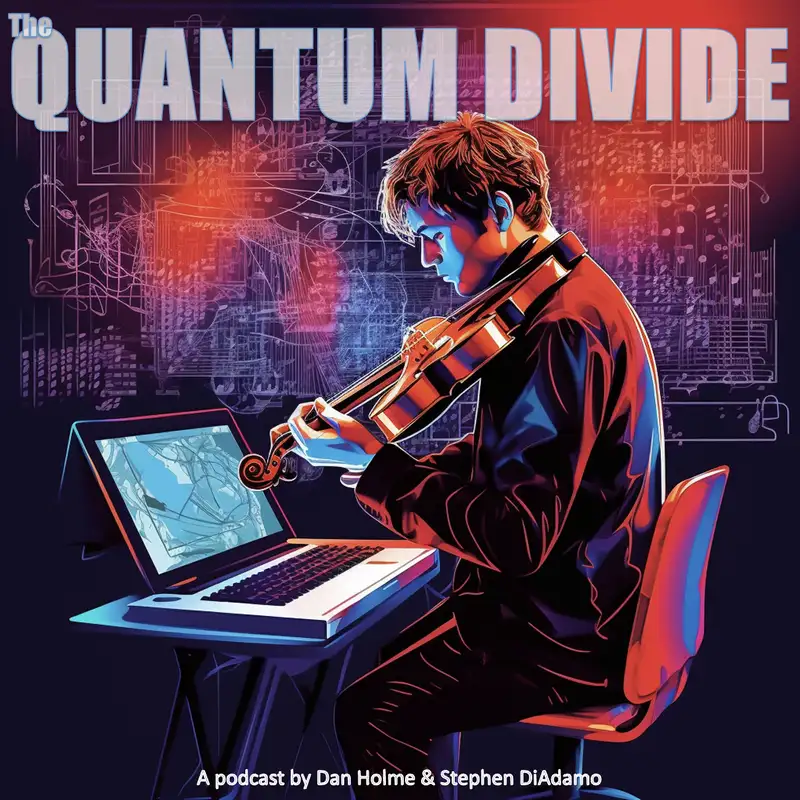