Transferring Quantum States
Dan: This is the quantum divide.
It's a podcast for people
curious about quantum networking.
What it means for the it industry
and what it might mean for them.
It's still early days for quantum,
but it's a very broad industry with
many different topics to understand.
The most imminent.
Our post quantum cryptography
and quantum key distribution.
Looking further ahead.
There are many academics building
ways to distribute content computers.
To leverage the power of
multiple machines over a network.
And how to manage quantum
state over that network.
Greetings I'm done home.
I'm a quantum curious technologist.
With no classical training
in quantum physics.
So basically that means.
I'll be the one asking
all the stupid questions.
For me, this podcast is a
vehicle to build my knowledge.
And I want to take you
on that journey with me.
Thankfully, I won't be producing
this podcast all on my own.
I'll be joined by my colleagues, Steve.
We want to bring you as many interesting
topics and discussions on quantum
networking that we can together.
Steve, why don't you introduce yourself?
Steve: Hello, I'm Steven dmo,
a research scientist at Cisco.
My background is in quantum
networks and communication.
I'll also be asking the stupid questions.
This podcast has a twofold benefit for me.
One, to stay up to date with
the current trends in quantum
networks and communication.
And two, to improve on being able to
digest the material for the interested
listeners, thanks for joining.
Dan: Okay, for this first episode,
just a couple of minutes to talk about
the vision really for this podcast.
Quantum networking for me
is such a fascinating topic.
I guess not only because of the
sci-fi field of the technology and
the way quantum mechanics works, but
also because computer networking in
general is a passion for a long time
and I feel like I'm coming home to
it, having been away for some time.
Albeit on a slightly alien planet.
So now onto the goal of this
podcast is to slowly educate
myself and you on everything that's
happening in quantum networking.
Initially, Steve and I will cover some
fundamentals, but when I say cover, I mean
discuss peppered with some mild confusion.
I would thank, we won't explain
the absolute basics, but we'll
start with quantum state.
We'll touch on quantum hardware for
networking, direct transmission,
entanglement, distribution, swapping,
and whatever else we come across.
We'll make sure we go into integration
with classical networking, all
the cryptography topics like Q K
D and post quantum cryptography.
But ultimately we're gonna have
some fun with this initially, and
then eventually we'll start bringing
in a wide range of experts and
dabblers to paint a broader picture.
I would say the target audience
here is anybody working in quantum,
but also anybody working in it?
Okay, Steve, let's get started.
Steve: Yeah.
So the key point of the key thing to
do when you're moving quantum stays.
Okay, let's see.
So there's two ways to do it.
Like when, if you wanna bring a qubit
from Alice to Bob over some significant
distance, then there are two ways
to do that, and that's using this
transmission over the quantum channel.
So you just take the cubit, put it into
a fiber, send the cubit through the
fiber, and then it arrives at Bob site.
The problem with that, which I,
yeah, there's some pictures there
when you can't talk about pictures.
You can't show the, you
can talk about pictures.
You can't show the pictures in a
Dan: Yeah.
Steve: Yeah.
So unfortunately, but I
can explain that more.
So the problem with this is when you
transmit a quantum state, if that quantum
state is holding valuable information
and then you send it through the fiber,
you have not much control over keeping
that quantum state in the fiber.
So the quantum state can convert,
get kicked out of the fiber,
and it'll never make it to Bob.
And if you use a lot of resources
to pair the quantum state, then
you've lost something quite valuable.
So what people propose is, instead of
sending the qubit directly through the
fiber, because as the fiber increases
in length, it gets even more hard to do.
The direct transmission is
to use quantum teleportation.
So quantum teleportation is in.
Protocol, so to say that uses
Another part of quantum is quantum
mechanics is quantum entanglement.
So instead of sending the information
containing cubics over the fiber,
what you send is something that's less
valuable is half of an E P R pair.
So that e p R pair you can
generate over and over again.
It contains no information.
It's just a renewable resource, so to say.
You can just generate it repeatedly.
It contains information and if you
lose it, it doesn't really matter,
but if it makes it to Bob's size,
so if Alison Bob share the entangled
pair, then they can send the qubit
state the important qubit state using
the entanglement without risking
the loss of that qubit so they can,
Dan: Yeah, let me lemme take
a step back for a second.
First of all, the approach is
sending Quantum State over the
Quantum Channel using Photons.
How is, how, just for somebody that
knows about traditional networking
and optics, how is that different
to using light to carry traffic?
My understanding is that it's actually a
case of sending one photon at a time.
Is that right?
Steve: Yes, correct.
So when you send a classical
message, the intensity of those
photons, which means the quantity
of those photons, It is just higher.
When you go to the quantum level,
it's basically just filtering out all
of sorry, all the additional photons
until you're left with one, and that
photon will hold the quantum state.
it's, that's, there's a lot
of similarities with classical
communication and quantum communication
using photons fiber optics.
It's more or less.
The challenge of getting the
photons across the channel is
much harder in quantum because
you just have that one chance.
If that one photon and all the information
is in that one photon, but when you're
sending a zero state or one state
classically, you essentially just turn
on the intensity, make it high enough,
it can make it across the fiber.
At least some of the photons
will make it to the fiber.
And there's multiple copies of the
same state where in the quantum
case, you don't have that ability.
You have one chance.
Dan: And that's why they say The
sending the state is very lossy, right?
It's very lossy.
But because you've got the traditional
things like dispersion of lighting
and perhaps the distance the quality
of , and power of the optics and so
on, all of those things, which once a
link is tuned correctly in traditional
networking is sufficient enough to
send up to, 1, 10, 1400, whatever.
But so there is no way to try and
optimize that really, because you
have to send one at a time and have
a detective that's receiving the
same stream in the right order at.
Steve: Exactly.
So there's some exceptions.
There's some schemes to what they call a
quantum amplifier, but in general For an
arbitrary qubit, you can copy it and you
can't make any repetition of the state.
So you get one chance and that's all it.
If it's lost.
You can't recover it.
You can, you basically have to restart
from the beginning, so that's the problem.
But yeah, in terms of the classical
communication, you just, you have
much more power to work with.
You have repetition, you have copying,
you have much more to get that.
Information from point A to point B.
Dan: What would be the benefit of
the approach of using sending the
state over the quantum channel?
Steve: Sending the state directly over
the quantum channel is a lot easier than
establishing entangled pairs between
distancing parties, be mainly because
there's no quantum memory at the moment.
There exists some quantum memories.
They're not at the moment good
enough to do quantum teleportation.
In the lab, there's been demonstrations
of quantum teleportation, but in the
lab setting you have a lot more control
over what's happening in the network.
But yeah, that's the advantage
of using direct transmission is
you don't need quantum memories
and you don't need to establish
entangled pairs with high fidelity.
Those are easier to do sometimes,
like so distance in when it comes to
long distance, you have no option.
You have to use some form of
teleportation or quantum or repeater.
So for short distances, probably
direct transmission could, especially
for things like Q K D, quantum P
distribution, it's probably makes more
sense just to use direct transmission.
But if you're doing something like
distribute a quantum computing, then yeah.
I think I got a little
bit off topic there.
Dan: It's easily done.
Done.
Steve: why?
Why one or the other?
So direct transmission is
simpler to do in general.
Although you can't, it
has the finite distance.
If you want to exceed the finite
distance, you have no choice but use
quantum teleportation, at least in
the early stages of quantum networks.
Dan: okay.
So I'm still sticking on number one here.
Trying to take it slowly.
Sending the state over the quantum
channel, you would be sending qubits
with particular are they in a super
position as they go across the link
or are they sent with a particular
state to be read via the end?
Steve: So it depends on the
protocol that you're running.
So in general, the quantum state is
in any state, any superposition state.
It could be completely, it's
completely up to the protocol.
Yes, it could be in a super position,
but if you're doing something like
Q K D, okay, some of the states
are not in a super position,
but some of them are, depends.
There's so many factors, but in general
you can't make any assumption of what,
what's on, what's contained in that qubit.
So I would say yes, it's always
in a super position state,
cuz that's the safe assumption
Dan: So with Q KD as an example,
what would you be sending over
the link to make that work?
Because we're not talking
about entanglement yet, are we?
So that, that's gonna be a different
set of photos to be sent over the link.
So if you're not doing
entanglement what is it that you
would want send over the link?
Steve: So in the Q K D case, that's
why I like Q KD so much is because
it's like a stepping stone application.
It's not such an intense
application that we can't do it yet.
We definitely can do it now, but what's
contained in the QBI is classical
information encoded into the qubit.
So that means, it means
it's still a quantum state.
It's still a quantum piece of
information, but encoding it is
more it's almost a binary state.
You just have to perform binary encoding.
That kind of simplifies the encoding
process and the decoding process
because you can use things like a beam
splitter, which can direct the zero
state or the one state accordingly.
But what happens is in Q kd, especially
like BBB 84 for example, is the
simplest example to use, I think, is
you polarize the light in the one zero
state or you polarize in a horizontal
basis, which is the plus minus So it's
just using a superposition state or
a classical state, and you transmit
classical information in that way.
Dan: Okay.
Does it give you more options to encode
things in less packets or something?
Because you have more different things
you can represent on a single qubit.
Steve: And Q K D is
still representing bits.
So each photon contains one You
can't exceed one bit per qit.
That's like a Some capacity,
some theoretical capacity of much
information can encode and decode.
On one qubit, you can put infinite
amount of information on the qubit by
encoding, it's a completely continuous
block sphere, for example, but
extracting the information with one
qubit, you can't ex exceed one bit.
Dan: Yeah, that's a good point.
So my understanding about the blood sphere
is it's it's the wave function, right?
Which will collapse when when it's.
Observed into one particular state.
So how could you carry, maybe this
is a tangent, but how could you carry
infinite information if actually when you
look at it, it's only gonna be one bit?
Steve: It's, yeah, that's, you
could put, yeah, that's the thing.
Like for example, let's say you'd
have a thousand bits of information.
That thousand bits of information
can be put finite, decimal
number and then the blocks space,
continuous sphere as just a degree.
You can think of it as just pointing
in a globe or something, in a sphere.
So you can make that infinitely
precise and you can put the
information into that sphere.
yeah, I see you mean like the
information is physically there,
but getting it out is not possible.
And that's why like the
decoding is important.
You can't extract that information out.
So that's the problem.
It's, yeah.
So it is, it's, it depends
how you look at it.
So the information is physically
there, it out, you can't get it.
It's just, it's gone.
Dan: Okay.
I think
Steve: So you can encode it.
but you
Dan: I think I'm confused enough now
to to probably move on to the next one
before it gets even worse.
Quantum entanglement and teleportation.
I know that teleportation is
not like the traditional kind
of Star Trek type teleportation.
And what we're doing here is splitting
entangled pairs of photons, and
these entangled pairs are entangled
in such a way that an action on one.
results in, in same behavior in the
other because they are connected
through the wave function somehow.
The wave function of the universe
Steve: I think so this
wave function observation.
So when I hear wave function, I
think of quantum mechanics and
I think of the short equation.
And the short equation basically dictates
how the quantum state evolves with time.
When you think about quantum
computing, quantum communication,
generally you don't.
Want things to evolve with time, you want
them to evolve after you operate them.
So I like the connection between
wave function and block sphere wave
function would dictate how if you
take the system, you put it into an
environment and then you just observe it.
The wave function will determine how
that system evolves with Tide, with
respect to the environment that it's in.
So you can imagine, let's say
you take a qubit, You prepared in
a state, then you put it into a
magnetic field and then you watch it.
And how, what happens to that qubit in
the magnetic field is defined by the
wave function a particular point So
it's usually a time dependent thing.
So when I think about quantum
communication, I think we don't
want that quad of state to change.
When you encode something into the
qubit, you want it to stay that
way until it's recovered by Bob
or else the information changes.
Basically the, you get
noise in your decoding.
So I always try to picture things
in terms of just fixed Noiseless,
evolution of quantum states,
they only evolve when we a we.
Put them in through shine light at them
or somehow interact with the cubit.
That's the only time it changes.
So it's almost not time dependent.
It's like discrete, time dependent.
I dunno, but you only change when it only
changes state when we do something to it.
So that's I think, an easier
yeah, You have the cubit, it's
fixed until you operate on it.
Dan: Fun.
Steve: So it's entanglement
is, like you said, they're
correlated to photons, to cubits.
They're correlated in a way that if
you measure one of them, then there's
something happens to the other one.
Operating on them alone is not
enough to influence the other.
You have to make a measurement, and
when you measure, then it collapses
the quantum state into a subset of
the states and those subset states.
Actually influence, it forces the
other part of the entanglement to
collapse into one of those states?
Dan: Always identical.
The states, which are a
result after the measurement.
Steve: No, not always.
So there's what we call
maximally entangled states.
Maximally entangled means that
they're either completely correlated,
which means they're exactly the same
or they're anti-correlated, which
means they're exactly the opposite.
But it's not always necessary to
have maximally, entangle, state,
and therefore they don't always
have to be the same output.
And they only also don't have
to have the same patterns.
They don't have to be like
completely correlated or completely.
Antico is something in the middle too.
Dan: So how do you want to explain
the quantum entanglement in
your yeah, I, I know we're both
looking at a circuit diagram here.
Do you wanna describe that or yeah.
Steve: Yeah, so usually what we think
about when we talk about Quan protocols,
you usually want to use the maximally
entangled states that are either
completely correlated or anti-correlated.
The protocols can be modified accordingly.
It's not so important what flavor
they have, but usually we're talking
about the maximally entangled state,
which is in the zero 11 bell state.
That means like if you measure zero
on one, you'll get zero on the other.
For sure.
If on one, you get one on the other side
as well, like completely deterministically
after the measurement has been made.
And those are easy to work
with because then you can write
the math down much simpler.
And thinking about that perfect
correlation is a lot easier to use.
So in quantum teleportation,
we assume that it's that exact
state, the 0 0 1 1 state.
Yeah, so Quad teleportation uses that
state, and at least in principle,
in practice things are different.
You have noise and you somehow,
when you're distributing bell pairs,
you don't always get that state.
You could distribute a bell pair but not
get the 0 0 1 1 state anyways, divergent.
But in the circuit diagram, we are looking
at, we have a arbitrary quantum state.
And then we assume that there's this
bell pair that's already established
between the two communicating parties.
Then what we have, so that means
that Alice, who is descending, the
qubit, has her quantum state plus
one additional state, which is her
of the total entanglement state.
So it's an additional qubit.
So she has two cubits in total,
one containing her information
and one half of an entangled pair.
On Bob's side, he has the other half of
the entangled pair and he doesn't need
to So he only interacts with that qubit
when Alice specifies what to do with it.
So on Alice's side, she takes her qubit
and she performs a certain operation.
And that's a two qubit operation where
she interacts her information containing
qubit with her half of the entangled pair.
And then she has to perform
a measurement on her side.
Actually, she measures
both of those qu bits.
And that's because when you make the
measurement, then the system collapses.
Only when you make the measurement
does the system collapse,
and then she sends those.
So that's what Alice does.
And then now she has, because
she measured two qubits, she
has two bits of information and
those two bits of information she
has to classically send to Bob.
So that disappear classical transmission.
And Bob receives the two bits of
information and he can now do something
on his half of the entangled state,
which is still in a quantum state.
It's not measured.
He has to hold onto it in some memory.
And with those two bits of information, he
applies what we call like a corrections,
some kind of quantum correction.
And what happens after he applies
that correction is his half of the
entanglement is exactly in the state.
Of the information containing qubit
that Alice had on her side and that's
the quantum teleportation protocol.
So now he has a qubit.
It's not measured, he just
performs certain operations on it.
And now what information that qubit holds
is exactly the information that was held
by Alice's side, by Alice's information
contained And very importantly is Alice
no longer has access to any of her qubits.
They're gone.
Or at least the information
inside those cubits is gone.
So It's a physical, it's not a movement.
It's a transfer of information.
So the information is lost on Alice's
side, and it exists on Bob's side.
Dan: Yeah, it's fascinating.
It really is.
So you've got the no cloning, the,
Is what you're referring to there.
But the fact that you can essentially
send information across the optical link
without needing to send the information
across the optical link is wild.
It really is.
Steve: One thing though, is
there is one transmission made.
It's a classical transmission.
Like that's, I know what you mean.
Like it's a fascinating for sure,
you're sending quantum information.
Using classical transmission
that's more precise.
I'd say it's, but you still
have to make a transmission.
You still need some connection
between Alice and Bob.
Dan: Sure.
But the information that's encoded
across the entangled pair is not sent
across the classical connection, is
it?
It's more like a
controlled, the way I see.
It's like a controlled layer.
Steve: that's so that we
would rely on this scheme for.
Transmitting quantum information over
long distances simply because it's too
lossy to send the information containing
cubits directly through the fiber.
Especially when that cubit
is valuable, then it's really
costly to lose that qubit.
Dan: Yeah, and you're losing out
on our whole potential security.
Opportunity here, right?
And that, that the information that's
sent cross entangled pairs cannot
be captured in any way on route.
And I know that obviously we can't
break the laws of physics, so you can't
send it faster than speed of light.
But will it mean potentially that
in very big networks that there will
be quite large speed improvements
in the way data can be transmitted?
Because I'm assuming that the
entanglement that this, it's an instant.
When Bob looks at and acts on the,
on his entangled part of the pair
up there that he gets an instant
reaction from it to reveal the
same information that Alice has.
And that does sound like it's
faster than the speed of light, but
it's not because it's, the control
traffic has to be sent in advance.
Steve: Yeah, exactly.
So because those two bits of
classical information have to be
sent, over, using light speed.
There's no instant, there's an
instantaneous reaction to the
entangle pair, but Bob has no
idea that Alice measured anything
when Bob measures his qubit.
he doesn't know Alice measured hers as
well, there's nothing saying that his
qubit They he'll know that whatever
he measures Alice will have measured,
but that doesn't mean there's any
information encoded in that qubit.
Before any collapse has been
made, any been made is random
output 50 50 in a bell state.
So Bob will measure, he'll see a zero, and
he'll see a one with equal probability.
Alice is guaranteed to see the same
thing, but that just means we both
flip the coin and we saw the same
that's not message transmission.
So there's no Just because we both
have the same random output doesn't
mean I communicated anything to you.
But what's, what could be done is
you can use that as a it's not like
we can have faster communication,
but we can have faster strategizing.
So if we want to play a game together,
for example, and you say, I'll act, if
I measure zero, I'll act in this way.
And if you measure zero, you act in
that way and we can actually play.
We can actually improve in some
schemes, in some games using strategies.
And this is actually more interesting
to me that we can behave in synchronous
without communicating at all.
You can also do this classically,
but in some cases you can modify the
entanglement and actually improve on
the winning probability of some games.
So let me try to rephrase that so you
can imagine, let's say we're playing.
Strategy game.
We both are holding a coin.
So I flip a coin and I get heads and I
say, I'll move left if I and I'll move.
If I get tails and you do the same, or
maybe you do the opposite, and this way
we try to avoid collisions or something.
I don't know.
And you could do that classically,
and we don't have to communicate,
but if we have entanglement, we could
coordinate ourselves, say, I'll measure
my qubit, and you measure your qubit.
Or I'll apply as an operation on my cubit
and then measure it and you do the same.
In some cases you the game.
Yeah, that's not a very good But
you can play games with entanglement
and you can coordinate and win some
specific games with higher probability
than if you just had that coin,
Dan: now, whilst you are trying to explain
the interesting analogy, I am constantly
thinking about the application of this and
how it compares to classical networking.
And what, what applications are
there in classical networking
that this would either.
Improve or the equipment to, or act upon.
And I, it's difficult to think,
potentially control information
potentially decision making in the
network could use this somehow to
Who knows alter the way routing
protocols work or something.
But I would guess there's probably quite
a lot of the benefits that we don't
really know yet, or what they're gonna
be on, how this technology is gonna be
applied to changing the way information
is sent across networks and the internet.
Is there any research out there going
on at the moment that's looking at the.
The higher order applications of
this type, these types of things.
Steve: I know.
So in game theory, subset of
game theory, quantum game theory.
But going networks I know I've seen one
paper, something like five years ago
that talk about using entanglement to
manage congestion in classical networks.
And I haven't been able to find that
paper since, and I don't know if I'm
imagining it or not, but I swear I read
something about that at some point.
And it's essentially choosing paths
in a network using entanglement,
measuring the entanglement to just
make decisions on which path to take.
This way you can choose paths so that
you avoid congestion in the network.
But other than that, I haven't seen
too many results about, like thinking
about using entanglement in that way.
I know, an exhaustive list of scientific
outputs, it's, I think it's not
such a popular field at the moment.
Dan: Yeah, that's an interesting one.
This feels very top heavy too.
Put all of that type of technology
in just to optimize the routing in
that way and ensure that there's
perhaps no queuing, scheduling
happening, interfaces in the route.
But.
Steve: Maybe just one more point I
wanna make is, it's very important
to think about the things that can be
done better with quantum entanglement.
There's actually.
A lot of cases you think, okay,
we have this shared source of
randomness completely correlated.
But that doesn't mean
you have an advantage.
You can also take a ran pseudo
random number generator and plug
in the same seed, and we'll have
the correlated outputs as well.
You don't need entanglement to
have correlated random outputs.
So this is a very fine line
between quantum advantage and
not, and I think this also is why
it's not such a popular field.
It's very hard to find that advantage.
Anyway,
Dan: Yeah, no, this is gonna be
interesting, I think in this podcast this
part and one that we record going forward.
Ultimately we've we spent most of this
time talking about the very fundamentals
of how quantum states can be transferred.
But, there are many different
applications of that potentially,
and there's lots of different aspects
of it, such as the transmitters, the
receivers quantum memory you mentioned.
And the fact that needing to
use repeaters of some kind to.
Send quantum information.
I read something that that about a an
experiment where it actually proved
that quantum repeaters can work.
Something, I'm sorry, I don't have the
actual reference to me, but I'll put
it in the pod notes if I get a chance.
Yeah, and we've touched on the kind
of issues with direct transmission.
The fact that the links are very lossy.
Fiber optics obviously gives you a limited
amount of distance and obviously it's a
bit different when we get into space free
space networks like satellites and so on.
But perhaps that's, that's
definitely a topic for another time.
Is there anything else that you think
we need to add when it comes to the
fundamentals of how Quantum state is.
Transferred, I mean without getting
too much data into the physics.
Steve: I think that's mostly the.
That's the core of it, at least direct
transmission entanglement sw sorry.
Oh yeah, there's, I guess there's
one part yeah, if we go into the more
depths as like the quantum repeater
and what does the quantum repeater do?
That would be one part to touch on, I
think, because that's also, it's just
because you have the ability to do
teleportation doesn't mean you exceed
the limitations of fiber communication.
So you need, still need a repeater,
but teleportation basically enables
that repeater at least what we
call the first generation repeater.
Dan: Yeah, that's right.
Repeat is in the quantum world, are
not the same as fire or something that
is Receiving packets, deconstructing
them or reconstructing them or anything
because it, first of all, it can't
interfere with the photons and the qubits.
So it's using, these things
are using deportation, right?
To my understanding is to receive state
of incoming in cubics and transfer them
to different entangled pairs so that the
stake can then be passed on with that.
Let you know that's my
super high level view, but.
Steve: Yeah.
No, that's a good in, that's
a good interpretation.
It's simply just, it's exactly that.
Just teleportation chain, essentially.
Teleport, teleport, teleport.
And then eventually it
arrives where it needs to go.
But each of those teleportation
requires point to point
entanglement at least in some cases.
It depends how you're implementing
the swapping procedure.
But yeah, you could in theory
do it as a teleportation chain
or you can do this inte ent,
integrous swapping procedure that.
At the end of the procedure, the only, the
endpoints of the chain have entanglement
then you make teleportation directly.
So there's two ways to do it
somehow, but that's mostly it.
Dan: Yeah we're looking quite
forward into the future in being
able to do this type of thing.
Like I said I've saw an experiment
recently where they had enabled this
teleportation using a repeater, but
I guess that was over two links and
obviously in a very in a lab environment
basically, and using technology that's
that's built specifically for that task.
We're gonna be years and decades away
even, but for this to be applicable
in the real world, and I expect these
types of devices will end up looking
like perhaps transceivers or optics
that go in forwarding and information
management note of some kind.
Or you could call them routers,
but ultimately it depends on.
What is this?
They're doing
Steve: But yeah, no, it's, it makes sense.
Yeah.
These things are still completely,
it's mostly academic work.
I think we're some time away from it
deployed in practice, although it's
not, they're not stopping people though.
There's people putting these technologies
into metropolitan scale networks.
It's a push, like a lot of people thinking
forwardly, but also some groundwork to
Dan: Yeah, maybe just a couple of minutes
on, on, on tackling in the real world.
I'm aware of a couple of.
Partnerships that Cisco has with some
universities where some of these kind
of concepts are being tested in the
real world across metropolitan networks.
I'm aware of one in
Cambridge, for example.
Perhaps we can talk about
that in a separate call.
Are there any of us that you'd like
to highlight where quantum networking
is being tested in the real world,
and if So , which concepts that
we touched on today are relevant?
Steve: Yeah.
So generally these, what deployed
into metropolitan scales, either
Q K D as most commonly Q kd.
Actually, there hasn't really been a.
Any entanglement distribution networks
so far until recently, actually,
so the q kd ones are interesting
because it's a commercial product.
People want security and so these
are but , it's getting old, it's
not really, it's all the term.
in the young stage of quantum
networks, it's been done a few
times now and we've seen it.
It gets more advanced every
time, higher communication rates.
But for the first time, I think this
year we've seen a metropolitan scale
entanglement distribution network.
that's something to look at, I think.
And that's what deployed in New York.
I don't know much about it actually.
I need to do my own reading, but
I saw the highlights at least.
And so this network is different
because it's not doing Q K D, but it's
distributing entanglement as far as
Dan: So yeah, the thing with,
I'm translating what you said
there to, to me Q KD is not that
interesting to some people in the
field anymore because it's maturing.
You say That's right.
Obviously it's incredibly exciting in
the way that it's gonna be changing the
way we do cryptography and it's gonna
be important for ensuring we've got
quantum safe cryptography going forward.
Steve: Yeah, I think in general,
it's one of the, it's the most
mature, as you said, the most
mature helping and co communication.
It's gone through quite a few iterations,
definitely seen a lot of varieties of it.
So not only through fiber optics,
also through space communication near
distance, long distance, different
types of protocols, different designs
for the network for the Q K D.
Also, the classical parts have been
well established now, so not only
do you need to transmit the key.
Using the quantum states, but then you
need to extract the key efficiently
and then store the key efficiently.
So not only do you have, you
still have a key, you need to
keep that safe classically too.
So all the things like surrounding Q
K D have been not well established,
but they're much, much further
established than any other
quantum application for networks.
Yeah, I think yeah, I think Q KD is
a topic on, it's only, it's another,
Dan: Oh yes.
Okay.
Thank you Steve.
I think we'll wrap it up there.
There's just so many topics that we
could go into and I think we're gonna
have to end up recording quite a
few of these to to do them justice.
So thanks very much and
I'll speak to you next time.
Steve: My pleasure.
Dan: I'd like to take this moment to
thank you for listening to the podcast.
Quantum networking is such a broad domain
especially considering the breadth of
quantum physics and quantum computing all
as an undercurrent easily to get sucked
into So much is still in the research
realm which can make it really tough for
a curious it guy to know where to start.
So hit subscribe or follow me on your
podcast platform and I'll do my best
to bring you more prevalent topics
in the world of quantum networking.
Spread the word.
It would really help us out.
Creators and Guests
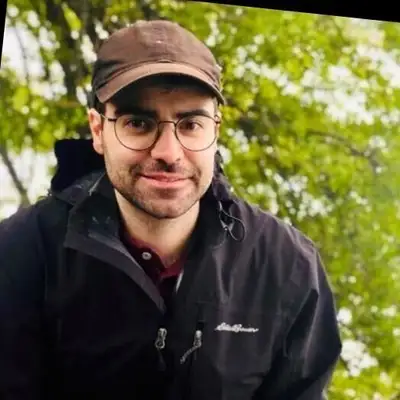
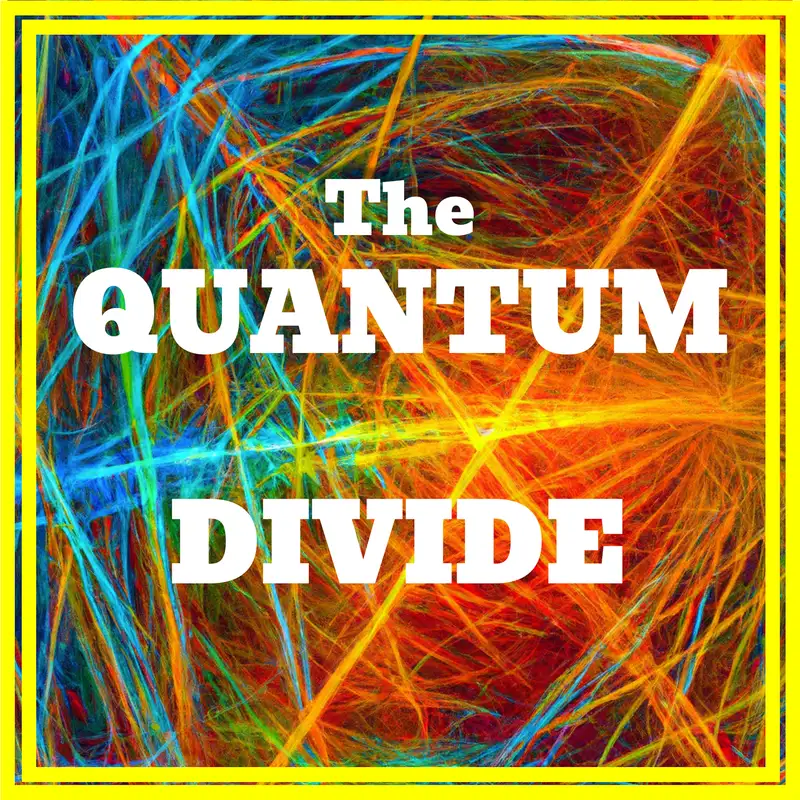